Introduction
Chemotherapy, irradiation and haematopoietic stem cell transplantation (HSCT) are now standard therapies in acute myeloid leukaemia (AML) [1]. Chemotherapies, anti-metabolites blocking DNA/RNA synthesis, have been a corner stone for three decades. However they have numerous side effects and limitations in efficacy [2]. HSCT is similarly challenged by toxicity and efficacy [3]. The overall 5-year survival from AML remains poor, particularly in adults and in the elderly [4]. Genomic and proteomic technologies have provided opportunities for development of targeted therapies through improved understanding of molecular biology, and better characterization of AML subgroups [5-7]. New agents are in phase I-III clinical trials. Several have been rejected as ineffective or unsafe but a small number have demonstrated potential [8]. However, none stand alone as mono-therapies or more effective than standard chemotherapy in low- and intermediate-risk patient groups, and continue to be assessed for adjuvant properties. This paper will review conventional treatments and their development, phase I-III clinical trials of new agents, novel pathways where future interventions may have therapeutic potential, and clinical trial assessment in AML.
Drug therapies
Chemotherapy
Chemotherapy protocols are divided in to two stages. The first, ‘Induction’, aims to reduce diseased cells to undetectable levels (complete remission (CR)). The second, ‘Consolidation’ (or post-remission), is the elimination of residual undetectable disease to achieve a cure [9-10]. In relapse treatment may revert to Induction, though there may be need to lower dosage depending on individual circumstance e.g., toxicity of previous therapy and level of morbidity [11]. HSCT might be undertaken if Induction chemotherapy fails or a patient relapses despite Consolidation therapy[12]. It may also be undertaken as first-line therapy alongside chemotherapy for patients with high-risk disease e.g., cytogenetic group, underlying myelodysplasia (MDS), or secondary and therapyrelated AML [13].
Successful intervention is hampered by cytogenetic heterogeneity, toxicity (Box 1), multi-drug resistance (MDR), and age [14]. Older patients (age >60) respond less well. In part this is due to the presence of co-morbidities but also greater association with MDS and secondary AML. In addition, clinicians have had a tendency to view therapy as palliative in older age groups, preferring to avoid toxicity, and studies have been biased by exclusion of these groups. In many respects it has been this poor outlook for elderly and secondary AML that has driven new agents through phase I and II trials recently. Although the majority of patients under 60 years reach CR after intensive chemotherapy[15-16], relapse free survival (RFS) is uncommon. The 10-year overall (OS) and event free (EFS) survival for children/adolescents after Induction therapy is 55- 65%. However, in adulthood only 20-40% of all patients gain disease-free survival (DFS) of >5 years from chemotherapy alone.
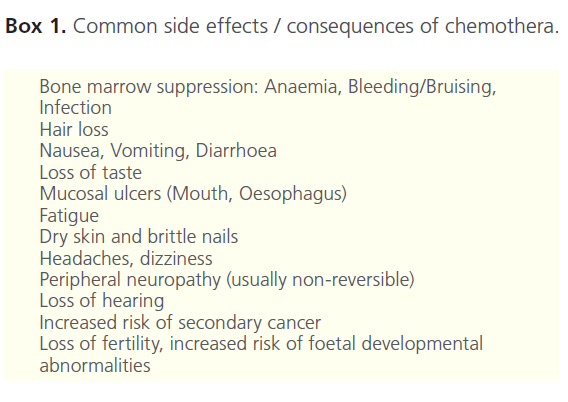
Box 1. Common side effects / consequences of chemothera.
Radiation therapy for AML is generally used only if there is central nervous system involvement and no response to systemic and/or intrathecal chemotherapy [17]. It may also be used in preparation for HSCT.
‘Old dogs, new tricks’
The focus of chemotherapy clinical trials research over the last 15 years has been to identify:
i. The most appropriate dose and frequency of administration,
ii. The efficacy of combination therapies,
iii. Whether certain agents are better than others by direct comparison,
iv. New formulations of established drugs (e.g., Liposomal drugs), and
iv. The value of adjunct therapies - facilitating effectiveness of chemotherapy and/or combating MDR.
Tables 1 (Induction) and Table 2 (Consolidation) are a synthesis of trial literature demonstrating the development of chemotherapies over the last decade and a half, with reference to differences between age groups, and to CR and OS. Various Consolidation strategies have been evaluated including intensive conventional chemotherapy, prolonged maintenance treatment, and high-dose therapy followed by autologous or allogeneic HSCT.
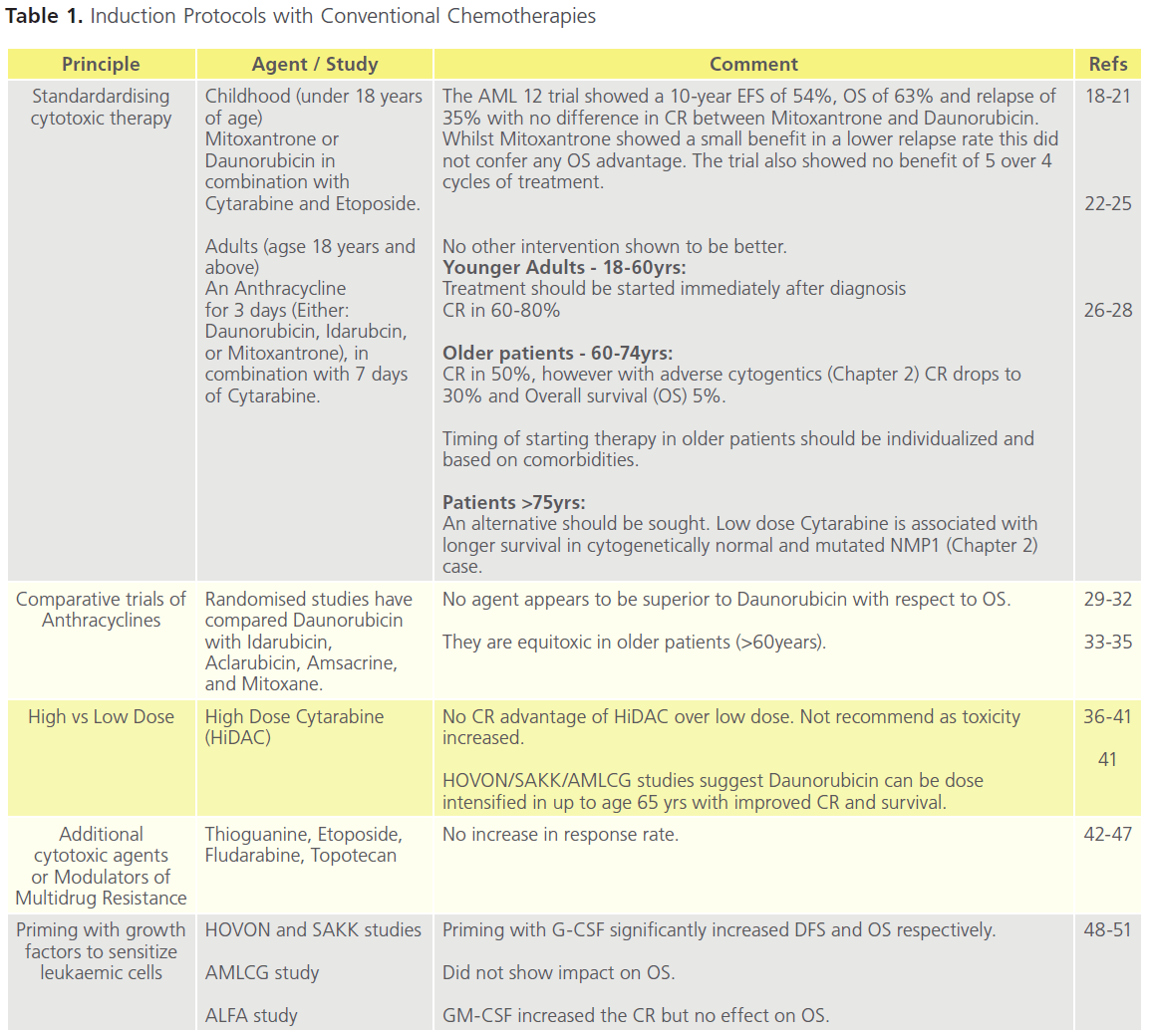
Table 1. Induction Protocols with Conventional Chemotherapies
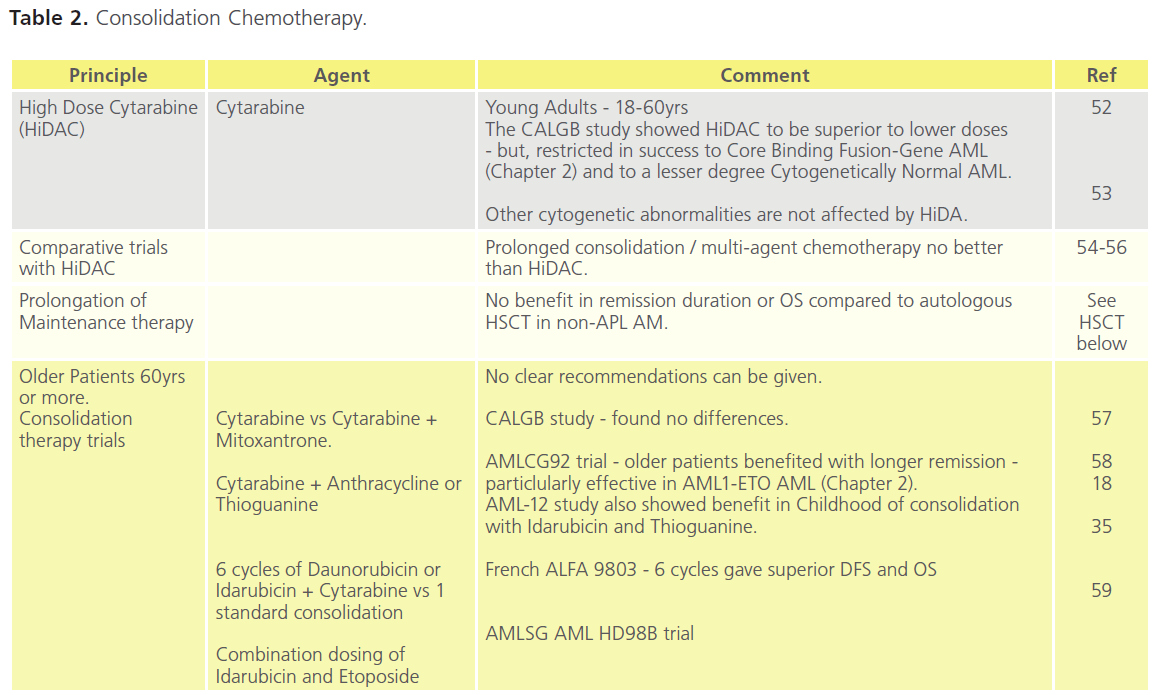
Table 2. Consolidation Chemotherapy.
The encapsulation of drugs in liposomes has led to new ways of more effectively delivering chemotherapy with a reduction in toxic side effects. Liposomal Daunorubicin has been shown in a Phase III trial to be as effective as normal Daunorubicin but better tolerated [60]. The agent CPX-351 is a liposmal fixed combination of Daunorubicin and Cytarabine. Recent Phase I/II trials suggest CPX-351 to have an acceptible safety profile for use in older and previously untreated patients [61,62]. Similarly, Elacytarabine, a derivative of Cytarabine but one that inhibits both DNA and RNA synthesis, has been demonstrated to be efficacious at least to the same degree as other agents, but with less toxicity in recent Phase II trials of patients in relapse requiring salvage therapy [63].
Given poor outcomes despite major advances in understanding best use of conventional chemotherapeutic agents, the need to develop novel therapies with different anti-leukaemic mechanisms is paramount. New agents entering the clinical arena include:
New Molecular Targeted Therapies
i. Monoclonal antibodies,
ii. Tyrosine kinase, and farnesyltransferase inhibitors,
iii. Cell growth blockers,
iv. Immunotherapies,
v. MDR1 inhibitors, and
vi. Peptide vaccines.
Table 3 and 4 outline trials in this area. In the majority of cases these trials have small numbers of patients and/or have selected for more complex disease and non-responders.
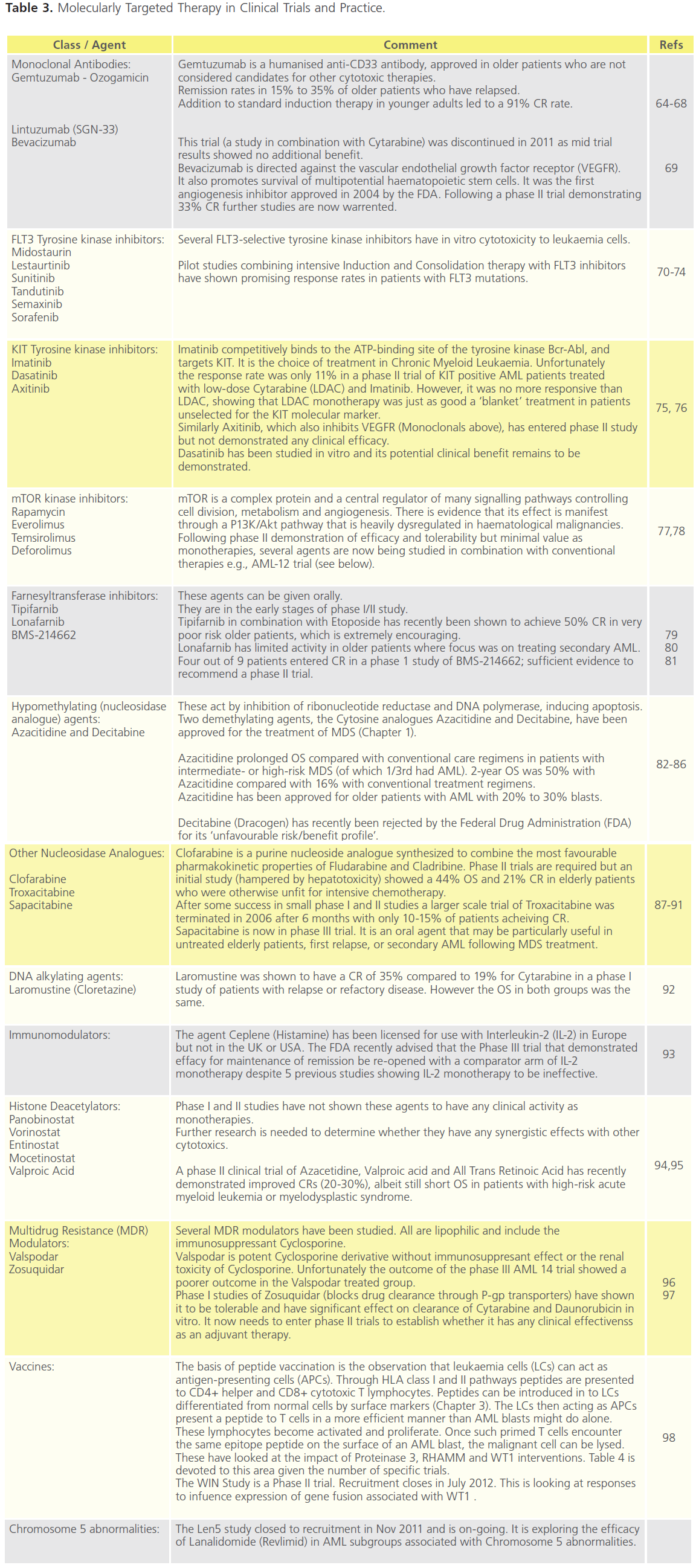
Table 3. Molecularly Targeted Therapy in Clinical Trials and Practice.
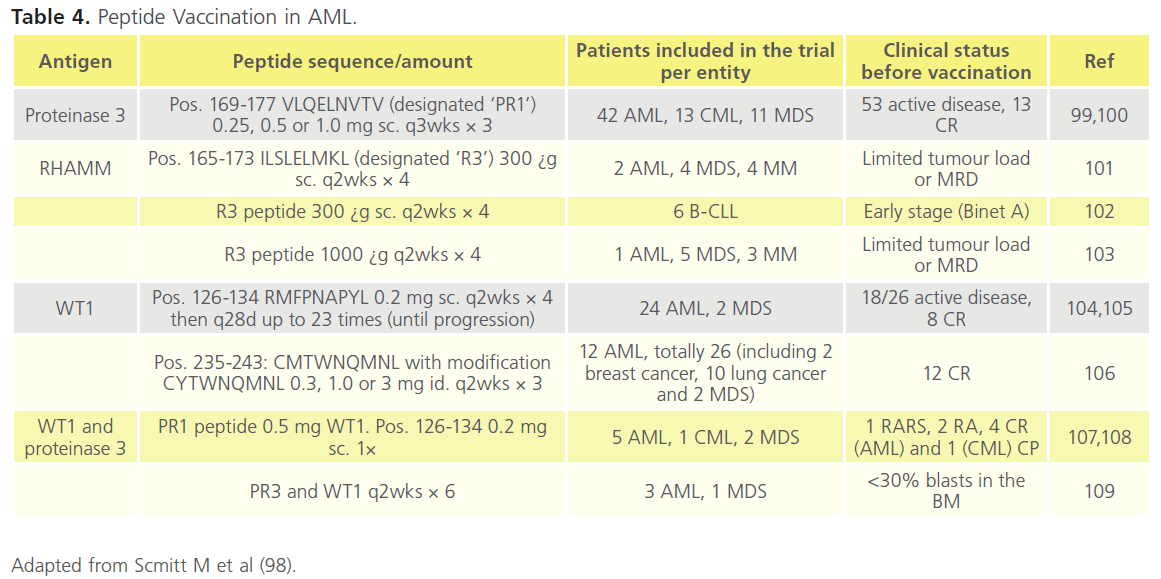
Table 4. Peptide Vaccination in AML.
On the whole the inhibition of deregulated transcriptional activity consequent on gene mutations has not led to therapeutic innovation; the exception to this is all-transretinoic acid and arsenic trioxide in APL.Inhibition of tyrosine kinase activity, nucleoside analogues, and monoclonal targeting of the antigen CD33 have demonstrated some success but none of these agents are superior to the combination Induction and Consolidation highlighted in Table 1 and 2.
Combinations of novel and current therapies are currently being explored in multi-faceted/multi-centred trials. The AML-17 (recruitment from 2002 to 2014) is one such phase III study looking at:
i. The best dose of Daunorubicin,
ii. CEP-701, a new FTL3 inhibitor,
iii. Everolimus (Afinitor) a signal transduction inhibitor that blocks the signalling protein mTOR,
iv. The comparison of 2 chemotherapy treatments before HSCT,
v. The comparison of 1 with 2 or more cycles of chemotherapy, and
vi. The role of Arsenic Trioxide in non-APL AML.
And in addition:
i. Clofarabine, a nucleoside analogue (see above), is being compared with other chemotherapies having been shown to have fewer side effects but similar efficacy to Fludarabine in the treatment for older patients considered unsuitable for induction chemotherapy, and
ii. Studies will continue to look at the role of Gemtuzumab.
There does not appear to be a revolutionary step change in drug therapy on the horizon in the management of AML. Attention is focused on synergistic effects of combining conventional with novel targeted agents. Though targeting of leukaemic stem cells (LSC) by, for example, receptor specific small molecules and peptide vaccines would appear a reasonable approach, the similarities between LSC and normal stem cells is also a challenge. Currently the targeting of LSCs remains relatively non-selective and requires simultaneous interventions.
Haematopoeitic stem cell transplantation
The developmental milestones in stem cell therapy (SCT) from the 1950s from preclinical trials to the successful application in human transplantation in the late 1970s are shown in Table 5. These laid the foundation for many areas of stem cell research, as well as current HSCT practices.
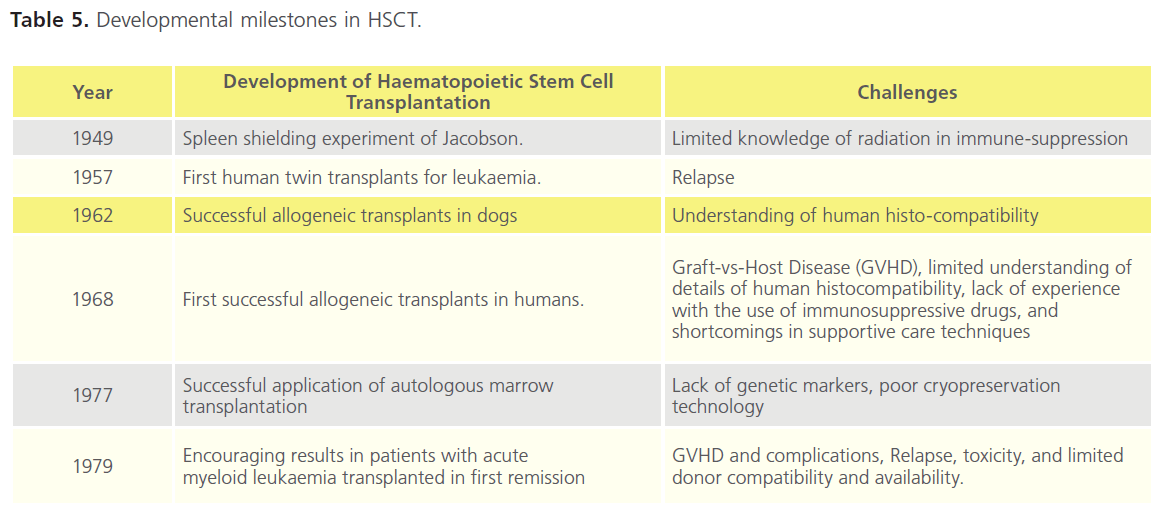
Table 5. Developmental milestones in HSCT.
Four sources of HSCT are available and each has its pros and cons (Table 6). The vast majority of clinical trials have been in allogenic and autologous stem cell transplants (SCT). Despite our learning of how best to use these therapies the challenges remain better techniques for cryopreservation, identification and classification of genetic markers, and understanding the influence of SNPs [3], non-HLA genetics, and cytokine genes [110-112].
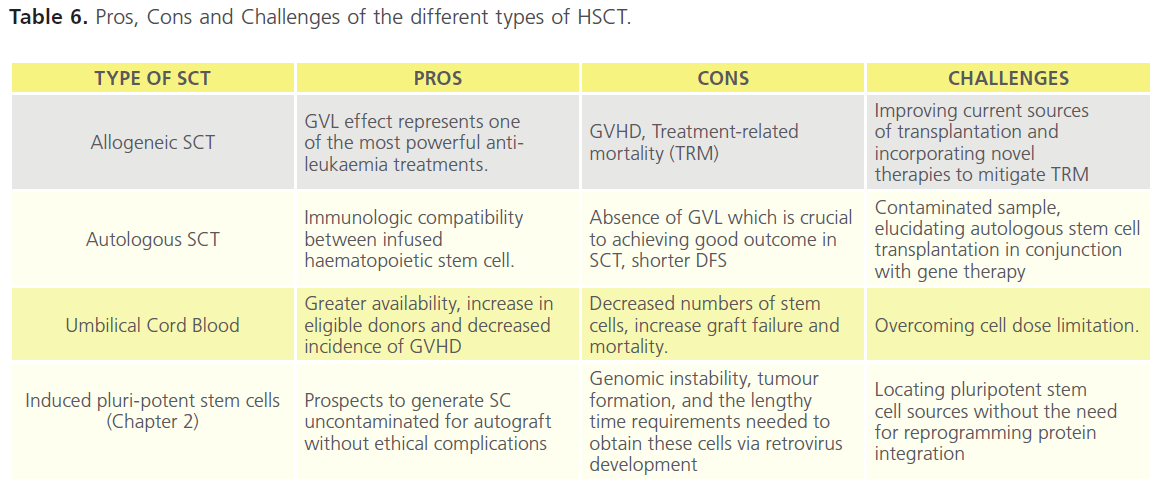
Table 6. Pros, Cons and Challenges of the different types of HSCT.
In patients with favourable- and intermediate-risk cytogenetics, autologous HSCT is an alternative Consolidation option to chemotherapy. It is not recommended in cases with highrisk cytogenetics [113-115]. There is no evidence that this approach gives a better outcome in general, however it may be of advantage in cytogenetically normal and tandem repeat subsets of AML [116]. The lowest relapse rates are observed following Consolidation with allogeneic HSCT. The benefit is in part attributable to a potent graft-versus-leukaemia (GVL) effect [117]. Meta-analyses of clinical trials comparing allogeneic HSCT versus Consolidation chemotherapies after first CR show a significant improvement in OS in intermediate- and high-risk AML [118-120]. Table 7 shows data from several studies in the 1990s. The DFS following Consolidation is in general superior for allogenic vs autologous HSCT, and for HSCT vs chemotherapy. However OS rates were not significantly different in a number of these studies. Data from the 2000s in childhood disease is more compelling for favourable outcome of DFS and OS after allogenic HSCT (Table 8).
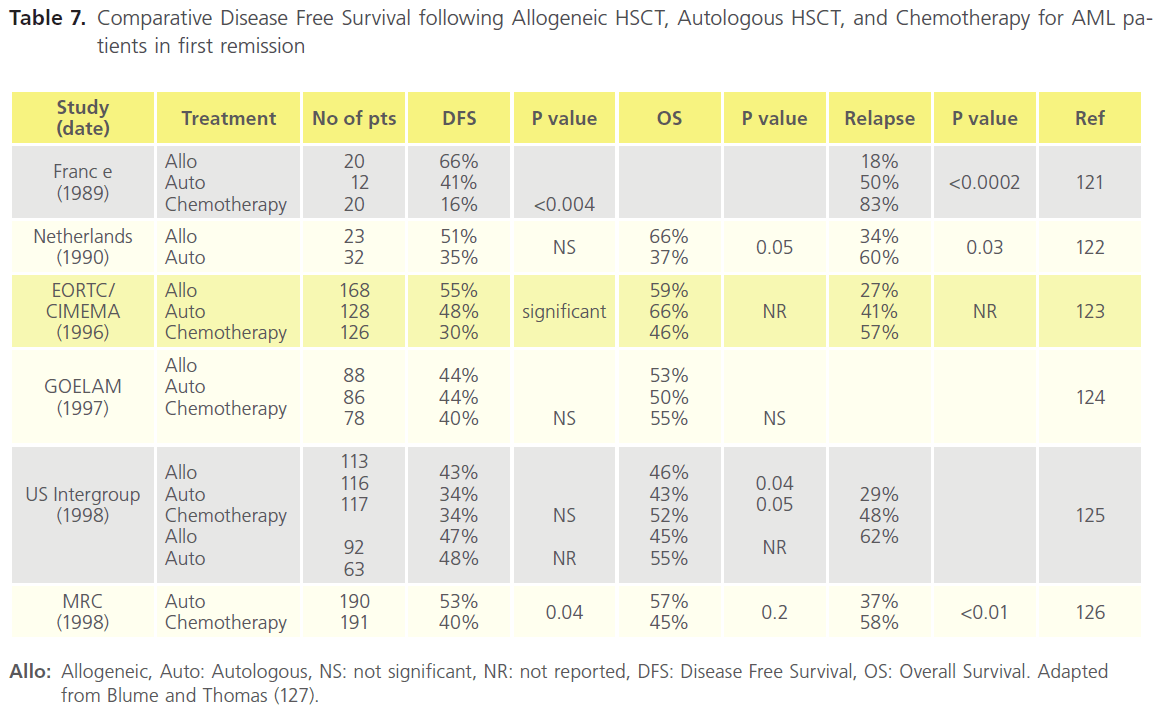
Table 7. Comparative Disease Free Survival following Allogeneic HSCT, Autologous HSCT, and Chemotherapy for AML patients in first remission
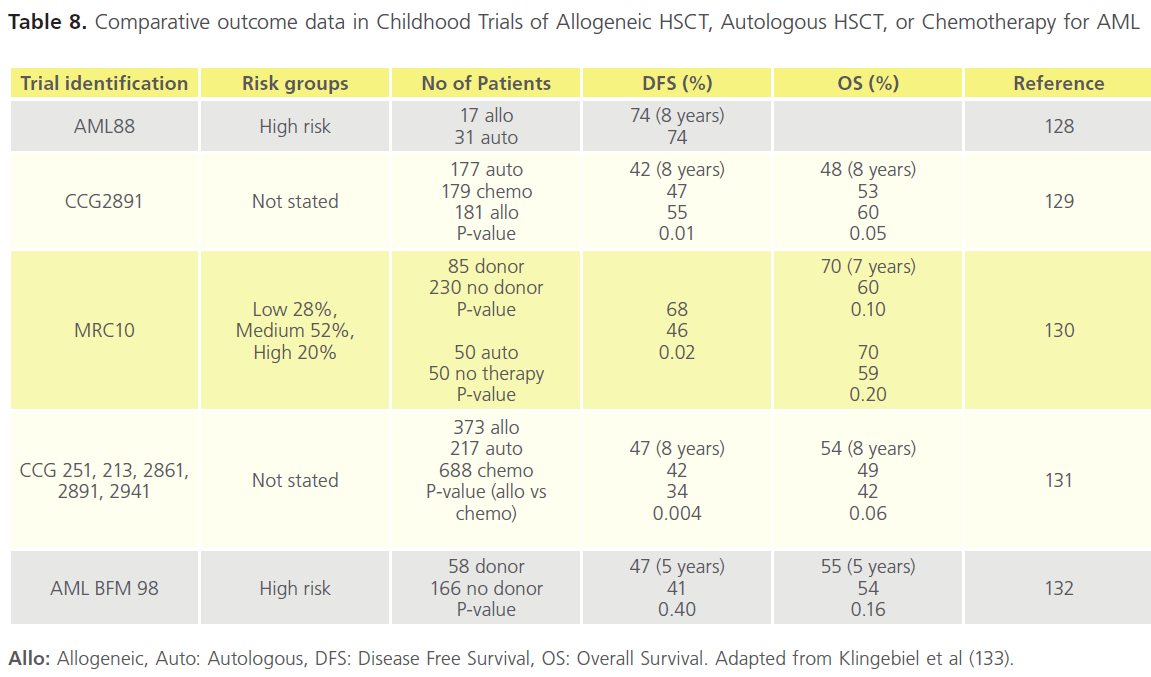
Table 8. Comparative outcome data in Childhood Trials of Allogeneic HSCT, Autologous HSCT, or Chemotherapy for AML
It is important to consider the risks of treatment-related mortality (TRM). These range between 15-50% and may out-weigh the benefits. These risks have been improved for older patients in particular by using reduced-intensity chemotherapy (RIC) regimes that are non-myeloablative. The outcomes are much more promising and comparable with younger cases [134,35],although relapse rates remain a challenge [136]. Data available from the European Group for Blood and Marrow Transplantation (EBMT) and the Centre for International Blood and Marrow Transplantation Research (CIBMTR) demonstrates that RIC regimens result in comparable outcomes across the adult age range (Table 9) [137]. As raised above for chemotherapy, data is difficult to interpret for older patients due to small patient numbers, heterogeneity, and selection bias. For these reasons prospective comparison of allogeneic HSCT from matched related and unrelated donors using RIC with conventional Consolidation therapy was launched in 2008 and as of December 2011 continues to recruit to this important clinical trial (ClinicalTrials. gov Identifier: NCT00766779). The use of RIC led to concern over graft versus host effects (Table 6) and has prompted research into immune-suppression studies to modulate GVL and GVH reactions [138]. Finally, research continues in to the benefit and most appropriate regimens using umbilical cord blood (Table 6) [139].
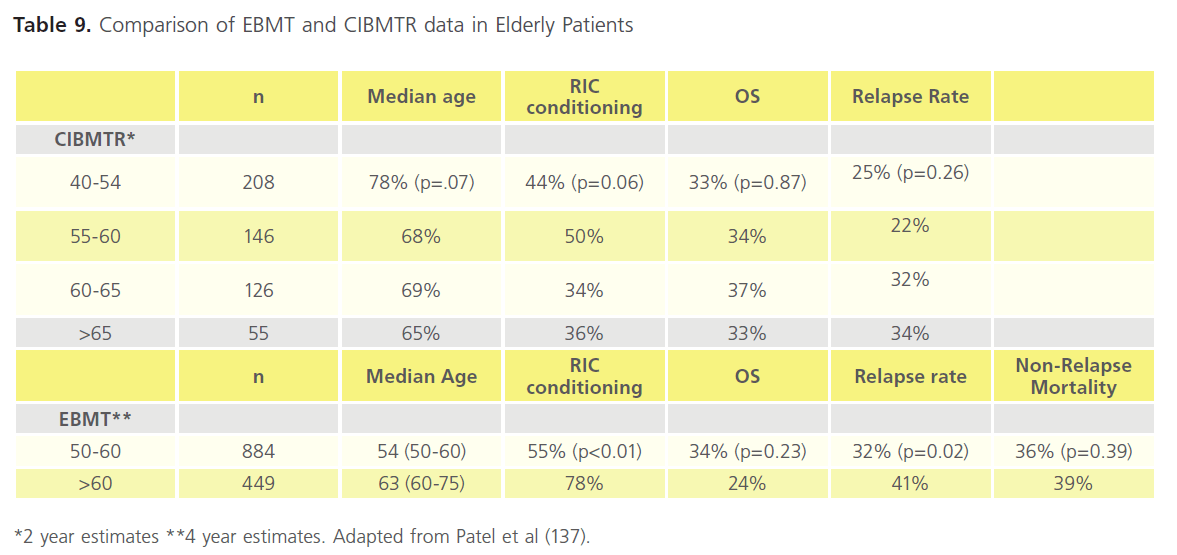
Table 9. Comparison of EBMT and CIBMTR data in Elderly Patients
Novel pathways and potential future agents
Several pathways and novel mechanisms of intervention are the focus of attention in current AML research.
AKT Inhibitors
As raised in Table 3 mTOR is a kinase involved in regulation of cell growth and proliferation. Signalling depends on its interaction through the PI3/Akt pathway [140]. Both PI3K and Akt are considered to be protooncogenes. Increased membrane expression of Akt is important in intiating malignancy. It also appears to confer resistance to apoptosis through the mitogen-activated protein kinase (MAPK) pathway [141]. As well as targeting mTOR (Table 3), Akt and MAPK inhibitors may represent new classes of drug. Perifosine is an Akt inhibitor and has shown preclinical activity against haematologic malignancies [142]. Phase I and II trials have been conducted in patients with solid tumours but not in leukaemias [143]. Alkylphosphocholines are lipophyillic drugs that have also been shown to modulate signal transduction by their interaction with c-myc, PI3-Akt, and MAPK pathways. Erufosine, an alkylphosphocholine, has anti-leukaemic properties that warrant further exploration [144].
RPRDX2
Studies have demonstrated that AML blasts exhibit significant lower levels of Histone H3 acetylation (H3Ac) compared to CD34+ progenitor cells. As a consequence it is suggested that a number of genes are epigenetically silenced or diminished in AML. Agrawal Singh et al [145] recently showed that Peroxiredoxin 2 (PRDX2) is a novel potential tumour suppressor gene in AML. H3Ac was decreased at the PRDX2 gene promoter in AML, and correlated with low mRNA and protein expression. Low protein expression of the antioxidantPRDX2gene was clinically associated with poor prognosis in AML. They identified PRDX2 acts as an inhibitor of myeloid cell growth by reducing levels of reactive oxygen species (ROS) generated in response to cytokines. Taken together, epigenome-wide analyses of H3Ac in AML, led to the identification of PRDX2 as an epigenetically silenced growth suppressor suggesting a possible role of ROS in the malignant phenotype in AML. This may be a pathway to explore in the application of Histone Deacetylator agents (Table 3).
TET2 mutation
Over the last 2-3 years mutations of Ten-Eleven Translocation 2 (TET2), have been found in various myeloid malignancies. The gene is associated with DNA methylation, mutations leading to inhibition or reduction in appropriate myeloid cell differentiation (Chapter 2) and appears to be a prognostic biomarker in AML associated with intermediate-risk cytogenetics [146-153].
In a study last year by Weissmann et al [154] 131 somatic TET2 mutations were identified in 87/318 (27.4%) patients, and in 30% of cases of normal karyotype AML versus 19% of abnormal karyotype. Mutations of TET2 were concomitantly observed with mutations in NPM1, FLT3-ITD, FLT3-TKD, JAK2, RUNX1, CEBPA, CBL and KRAS (Chapter 2). Patients tended to be of older age, with higher haemoglobin level, higher neutrophil and monocyte counts, and lower platelet count. Similar mutational associations were identified by Chou et al [155]. Survival analyses (restricted to the normal karyotype population (n=165)) in Weissmann’ study showed inferior EFS in the presence of TET2 mutations.
In two other studies, one retrospective [156] the other prospective [157] the presence of TET2 mutations did not appear to influence CR or OS after standard therapy. There has also been one clinical study in higher risk MDS and AML with low blast count, where TET2 status was observed to be a genetic predictor of response to Azacitidine, independently of karyotype [141]. Further clinical studies with such hypomethylating agents are warranted.
DNMT3A mutation
DNMT3A mutations are observed in up to 22% of AML patients and appear more prevalent in the intermediate-risk groups, and especially of normal karyotype [158]. The mutations are strongly associated with poor prognosis [159-161], and like TET2 are associated with decreased DNA methylation and promotion of cell differentiation. DNMT3A forms a complex with transcription factors like histone methyltransferase and histone deacetylase [162,163]. Novel DNA methyltransferase and histone deacetylase inhibitors can reverse the methylomic phenotype of myeloid blasts (Table 3 Nucleosidase and Histone Deacetylase Drugs).During therapy, early platelet response and demethylation of the FZD9, ALOX12, HPN, and CALCA genes were associated with clinical response. Epigenetic modulation deserves prospective comparisons with conventional care in patients with high-risk AML, at least in those presenting previously untreated disease and low blast count.
Trial methodology
Pre-clinical
In vitro studies of cultured native AML cell lines and blasts have remarkably contributed to our current understanding on the pathogenesis of AML (Table 10). Well-characterised serum-free in vitro conditions are now used in experimental studies of AML, facilitating comparisons between different experiments. Assays for characterisation of AML progenitor subsets such as suspension cultures, colony assays, long-term in vitro culture, xenotransplantation in immunocompromised mice, as well as, AML cell lines as experimental models have been used to increase our knowledge on pathogenesis of AML [164]. Furthermore, biomarker studies suggest that the in vitro growth characteristics of AML blasts assayed by shortterm culture of the total native populations can be used as a predictor of prognosis after intensive chemotherapy. In vitro assays may be used for more accurate identification of prognostic parameters and for creation of a basis for the development of simplified laboratory techniques suitable for routine evaluation of patients undergoing risk-adapted therapy [164].
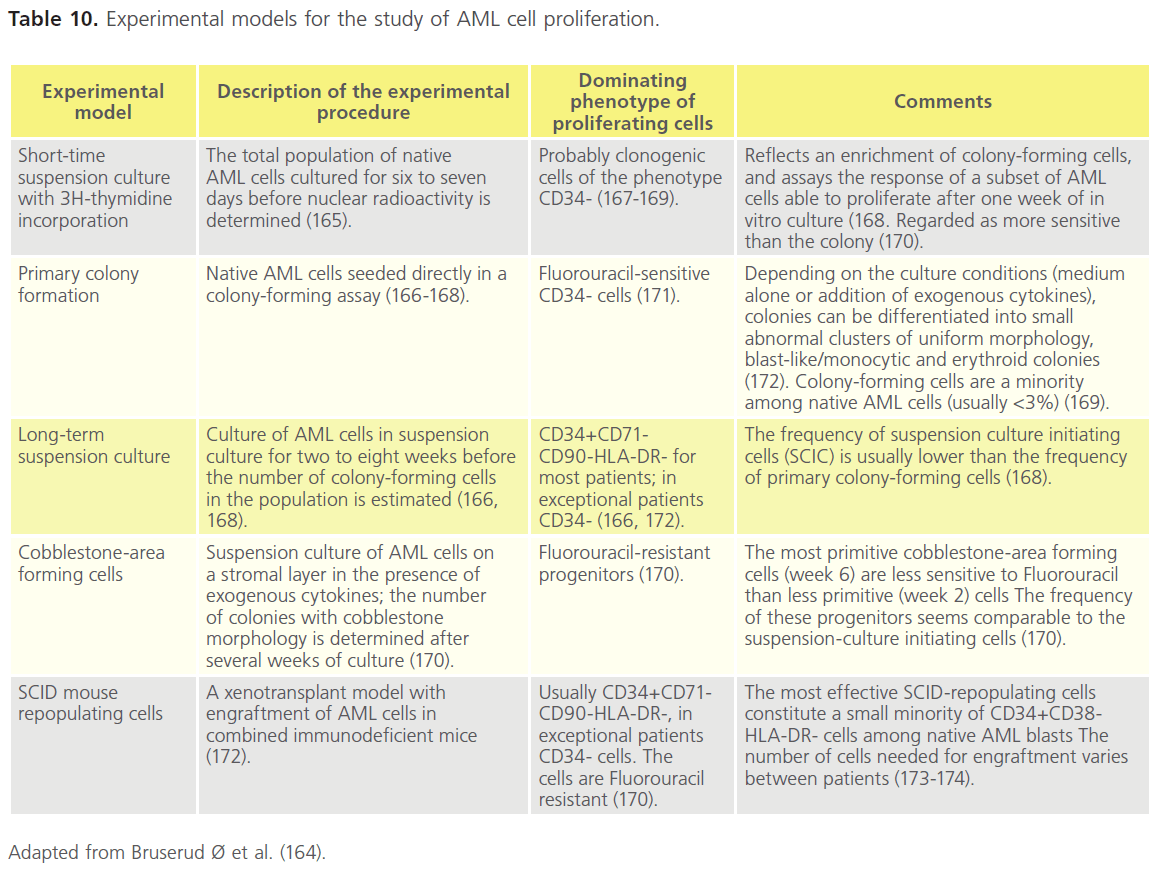
Table 10. Experimental models for the study of AML cell proliferation.
Clinical
Drug development processes are lengthy and costly. While the phase I-III sequence of clinical drug testing has remained intact for decades, it appears inherently inefficient and the high frequency of false-positive results obtained in phase II studies constitutes a significant scientific concern [175- 180]. The sequential trial scheme puts major emphasis on such studies because they typically inform the decision to proceed to a phase III evaluation [175]. Strategies to mitigate shortcomings caused by lack of control groups, patient heterogeneity, selection bias, and choice of end points and strategies for streamlining trial design have been suggested. Such enhancements would among others encompass larger phase II studies, inclusion of (preferably randomised) controls, consideration of integrated phase 2/3 studies, accounting for patient heterogeneity even in small randomised studies, provision of information about the number of patients available for study vs. those actually treated, and avoidance of unvalidated alternate endpoints and premature publication (Table 11) [175].
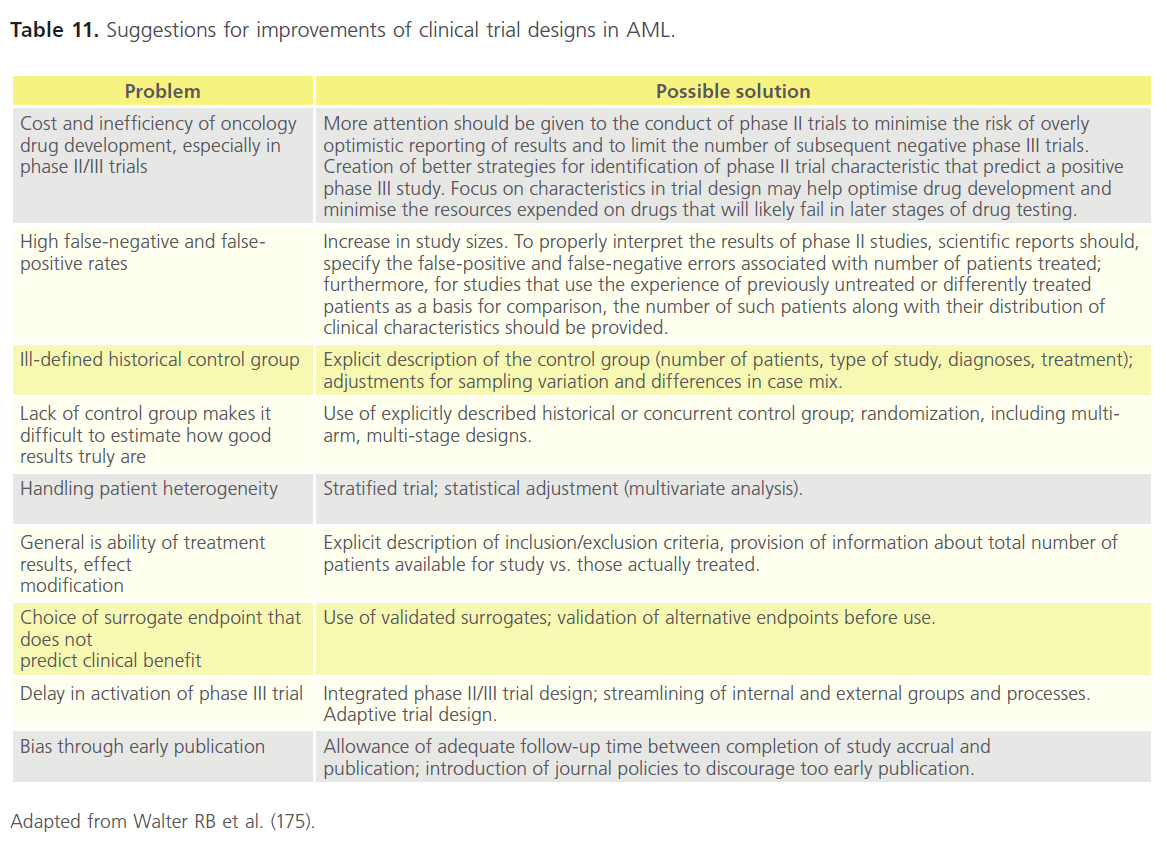
Table 11. Suggestions for improvements of clinical trial designs in AML.
Phase I trials often provide novel agents to patients with relapsed and refractory disease [181]. It has been argued that noncytotoxic, molecularly targeted agents have not been very successful in this setting. Thus, signals of their true biologic efficacy may be missed and consequently potentially useful agents seem fail to demonstrate a signal of efficacy in the phase I setting. It has been proposed that at least some of these compounds should be considered ins tead in trials to prolong response duration [181].
Phase II trials in AML are usually small-scale and may give misleading efficacy signs [175, 166]. Due to the heterogeneity of the disease, subset analyses based at least on age, performance status, cytogenetics, and molecular features are necessary [175]. However, these are meaningless when the total group includes a small number of patients [181]. Consequently, there is increasing support for randomised phase II trials strategies planned to quickly compare new treatments with existing standards using as few patients as possible and to proceed only with those that meet predetermined efficacy benchmarks [181].
Phase III trials in AML are often slow, expensive to complete and, regrettably, often resulting in minor improvements. It has also become increasingly difficult to determine a feasible control group for new phase 3 trials due to the large number of molecularly and clinically defined subgroups. Further enhancements in the molecular characterisation of AML could allow the identification of more homogeneous treatment cohorts and tailored therapeutics [181].
Translational strategies to accelerate drug development
• Focusing on development of existing drugs in addition to searching for new ones. Due to the heterogeneous pathogenesis and molecular genetics of AML, tailored, personalised treatment based on the specific biologic features of the leukemic cells should be the objective. This also indicates that combination therapy is likely to remain superior to any single compound [181]. One interesting suggestion is to use the gene-expression signature generated from drugs that effectively ablate LSCs to study publicly available databases for other similar signatures [182]. In the case of “off-patent agents” , this could possibly al-low existing agents with well-identified clinical profiles and easy availability, to rapidly lead into AML treatments [181].
• Increasing participation in clinical trials. Less than 5% of adult cancer patients in US participate in clinical trials, contrary to 60% of paediatric cancer patients [181,183]. Recently, a French survey reported that 25% of AML patients among 1066 adults with AML were enrolled in clinical trials [181,184]. Physician and patient education about clinical trials should be enhanced and collaboration between academic centers and cooperative groups should be improved [181]. Increasing accrual in clinical trials is vital, as there the traditional phase I-III drug-development paradigm seems ineffective in this disease [175,181].
• Improving of safety and efficiency. Implementing biomarkers in clinical trials may improve decision-making in drug development process [185]. Biomarkers predicting therapeutic response enable the selection of patients most likely to have positive treatment outcomes with a particular oncologic therapy. Predictive pharmacogenomic biomarkers enabling selective treatment, are likely to become increasingly common in future therapies [186]. Biomarkers predicting the safety of a compound are highly valuable for preclinical testing, or early clinical studies. Microdosing studies could be used for improving safety when evaluating drug candidates at early stage development. Adaptive trial designs in AML studies could improve safety and efficacy by providing opportunities to make changes to a study in response to accumulating data whilst maintaining the trial’s integrity and validity.
Conclusion
AML is characterised by a multitude of chromosomal abnormalities and gene mutations, which translate to marked differences in responses and survival following chemotherapy, radiotherapy and HSCT. These chromosomal and genetic abnormalities make the treatment of AML challenging. The limit of acceptable toxicity for standard chemotherapeutic drugs used in AML therapy has been reached. A detailed understanding of the molecular changes associated with chromosomal and genetic abnormalities is necessary to pilot new therapy design. Although several deregulated proteins and genes have been identified, their diversity among AML patients have made it difficult to identify a single substance that can hit these diverse targets . New agents have shown promise but there remains a huge need to be met for effective and targeted therapies to be successful.
5834
References
- Thomas ED, Buckner CD, Banaji M et al.: One hundred patients with acute leukemia treated by chemotherapy, total body irradiation, and allogeneic marrow transplantation. Blood .1977;49:511-533.
- Peters GJ, van der Wilt CL, van Moorsel CJ, Kroep JR, Bergman AM, Ackland SP. Basis for effective combination cancer chemotherapy with antimetabolites. Pharmacol Ther. 2000;87(2-3):227-53.
- Faduola P.Stem cell transplantation in acute myeloid leukemia; history, drivers and challenges.Int J Biol Med Res. 2012; 3(3): 2132-2137
- Kaspers, G. & Creutzig, U. Pediatric AML: long term results of clinical trials from 13 study groups worldwide. Leukemia.2005;19:2025-146
- Foran, J.M. New prognostic markers in acute myeloid leukemia: perspective from the clinic. Hematology Am Soc Hematol Educ Program, 2010, pp47-55.
- Godley, L.A., Cunningham, J., Dolan, M.E., et al. An integrated genomic approach to the assessment and treatment of acute myeloid leukemia. Semin Oncol.2011; 38(2):215-24.
- Zmorzyski, S., Filip, A.A., Koczkodaj, D. & Michalak, M. Molecular and cytogenetic prognostic factors in acute myeloid leukemia (AML). Postepy Hig Med Dosw,2011;21(65):158-66
- (160)Walter RB, Appelbaum FR, Tallman MS et al. Shortcomings in the clinical evaluation of new drugs: acute myeloid leukemia as paradigm. Blood. 2010;116(14):2420-28
- Lobo, P.J., Powles, R.L., Hanrahan, A. & Reynold, D.K. (1991). Acute myeloblastic leukaemia - a model for assessing value for money for new treatment programmes. BMJ, 302(6772):323-26.
- Dufoir, T., Saux, M.C., Terraza, B., et al. Comparative cost of allogeneic or autologous bone marrow transplantation and chemotherapy in patients with acute myeloid leukaemia in first remission. Bone Marrow Transplant.1992;10(4):323-29.
- Creutzig, U., & Kaspers, G.J.L. (. Editorial: Pediatric acute myeloid leukemia: international progress and future directions. Leukemia.2005;19:2025-2029
- Bensinger WI, Weaver CH, Appelbaum FR, et al. Transplantation of allogeneic peripheral blood stem cells mobilized by recombinant human granulocyte colonystimulating factor. Blood. 1995; 85(6):1655- 1658.
- Ljungman P, Bregni M, Brune M, et al. Allogeneic and autologous transplantation for haematological diseases, solid tumours and immune disorders: current practice in Europe 2009. Bone Marrow Transplant. 2010; 45(2):219-234
- Nataliya Kuptsova,Kenneth J. Kopecky,John Godwin,Jeanne Anderson,Ashraful Hoque,Cheryl L. Willman,Marilyn L. Slovak,and Christine B. Ambrosone. Polymorphisms in DNA repair genes and therapeutic outcomes of AML patientsfrom SWOG clinical trials. Blood. 2007 109: 3936-3944
- Buccisano F, Maurillo L, Gattei V, et al. The kinetics of reduction of minimal residual disease impacts on duration of response and survival of patients with acute myeloid leukemia. Leukemia.2006;20(10):1783-1789.
- Maurillo L, Buccisano F, Spagnoli A, et al. Monitoring of minimal residual disease in adult acute myeloid leukemia using peripheral blood as an alternative source to bone marrow. Haematologica. 2007;92(5):605-611
- Castagnola C, Nozza A, Corso A, Bernasconi C.The value of combination therapy in adult Acute Myeloid Leukemia with central nervous system involvement.Haematologica 1997; 82:577-580
- Gibson BE, Webb DK, Howman AJ, et al. Results of a randomized trial in children with acute myeloid leukaemia: medical research council AML 12 trial. Br J Haematol. 2011;155(3):366-76.
- Sekeres MA, Elson P, Kalaycio ME, et al. Time from diagnosis to treatment initiation predicts survival in younger, but not older, acute myeloid leukemia patients. Blood. 2009;113(1):28-36.
- Löwenberg B, Griffin JD, Tallman MS. Acute myeloid leukemia and acute promyelocytic leukemia.Hematology Am Soc Hematol Educ Program. 2003:82-101.
- Estey E, Döhner H. Acute myeloid leukaemia. Lancet. 2006;368(9550):1894-1907.
- Appelbaum FR, Gundacker H, Head DR, et al.Age and acute myeloid leukemia. Blood. 2006;107(9):3481-5.
- Estey E. Acute myeloid leukemia and myelodysplastic syndromes in older patients. J Clin Oncol. 2007;25(14):1908-15.
- Farag SS, Archer KJ, Mrózek K, et al. Pretreatment cytogenetics add to other prognostic factors predicting complete remission and longterm outcome in patients 60 years of age or older with acute myeloid leukemia: results from Cancer and Leukemia Group B 8461. Blood. 2006;108(1):63-73.
- Fröhling S, Schlenk RF, Kayser S, et al. Cytogenetics and age are the main determinants of outcome in intensively treated acute myeloid leukemia patients older than 60 years: results from AMLSG trial AML HD98-B. Blood. 2006;108(10):3280-38.
- Burnett AK, Milligan D, Prentice AG, et al. A comparison of low-dose cytarabine and hydroxyurea with or without all-trans retinoic acid for acute myeloid leukemia and high-risk myelodysplastic syndrome in patients not considered fit for intensive treatment. Cancer. 2007;109(6):1114-24.
- Fenaux P, Lai JL, Gardin C, Bauters F. Cytogenetics are a predictive factor of response to low dose Ara-C in acute myelogenous leukemia (AML) in the elderly [letter]. Leukemia.1990;4(4):312.
- Sekeres MA, Stone RM, Zahrieh D, et al. Decision-making and quality of life in older adults with acute myeloid leukemia or advanced myelodysplastic syndrome. Leukemia. 2004;18(4):809-16.
- Vogler WR, Velez-Garcia E, Weiner RS, et al. A phase III trial comparing idarubicin and daunorubicin in combination with cytarabine in acute myelogenous leukemia: a Southeastern Cancer Study Group study. J Clin Oncol.1992;10(7):1103-11.
- Hansen OP, Pedersen-Bjergaard J, Ellegaard J, et al. Aclarubicin plus cytosine arabinoside versus daunorubicin plus cytosine arabinoside in previously untreated patients with acute myeloid leukemia: a Danish national phase III trial. Leukemia. 1991;5(6):510-6.
- Berman E, Arlin ZA, Gaynor J, et al. Comparative trial of cytarabine and thioguanine in combination with amsacrine or daunorubicin in patients with untreated acute nonlymphocytic leukemia: results of the L-16M protocol. Leukemia. 1989;3(2):115-21.
- Arlin Z, Case DC Jr., Moore J, et al. Randomized multicenter trial of cytosine arabinoside with mitoxantrone or daunorubicin in previously untreated adult patients with acute nonlymphocytic leukemia (ANLL). Leukemia. 1990;4(3):177-83.
- Rowe JM, Neuberg D, Friedenberg W, et al. A phase 3 study of three induction regimens and of priming with GM-CSF in older adults with acute myeloid leukemia: a trial by the Eastern Cooperative Oncology Group. Blood. 2004;103(2):479-85.
- Anderson JE, Kopecky KJ, Willman CL, et al. Outcome after induction chemotherapy for older patients with acute myeloid leukemia is not improved with mitoxantrone and etoposide compared to cytarabine and daunorubicin: a Southwest Oncology Group study. Blood. 2002;100(12):3869-76.
- Gardin C, Turlure P, Fagot T, et al. Post-remission treatment of elderly patients with acute myeloid leukemia in first complete remission after intensive induction chemotherapy: results of the multicenter randomized Acute Leukemia French Association (ALFA) 9803 trial. Blood. 2007;109(12):5129-35.
- Weick JK, Kopecky KJ, Appelbaum FR, et al. A randomized investigation of high-dose versus standard-dose cytosine arabinoside with daunorubicin in patients with previously untreated acute myeloid leukemia: a Southwest Oncology Group study. Blood. 1996;88(8):2841-51.
- Bishop JF, Matthews JP, Young GA, et al. Randomized study of high-dose cytarabine in induction in acute myeloid leukemia. Blood. 1996;87(5):1710-7.
- Cassileth PA, Lee SJ, Litzow MR, et al. Intensified induction chemotherapy in adult acute myeloid leukemia followed by highdose chemotherapy and autologous peripheral blood stem cell transplantation: an Eastern Cooperative Oncology Group trial (E4995). Leuk Lymphoma. 2005;46(1):55-61.
- Petersdorf SH, Rankin C, Head DR, et al. Phase II evaluation of an intensified induction therapy with standard daunomycin and cytarabine followed by high dose cytarabine for adults with previously untreated acute myeloid leukemia: a Southwest Oncology Group study (SWOG-9500). Am J Hematol. 2007;82(12):1056-62.
- Büchner T, Berdel WE, Schoch C, et al.Double induction containing either two courses or one course of high-dose cytarabine plus mitoxantrone and post-remission therapy by either autologous stemcell transplantation or by prolonged maintenance for acute myeloid leukemia. J Clin Oncol. 2006;24(16):2480-9.
- Löwenberg B, Ossenkoppele GJ, van Putten W, et al. High-dose daunorubicin in older patients with acute myeloid leukemia. N Engl J Med. 2009;361(13):1235-48.
- Bishop JF, Lowenthal RM, Joshua D, et al. Etoposide in acute nonlymphocytic leukemia. Blood.1990;75(1):27-32.
- Hann IM, Stevens RF, Goldstone AH, et al. Randomized comparison of DAT versus ADE as induction chemotherapy in children and younger adults with acute myeloid leukemia. Results of the Medical Research Council’s 10th AML trial (MRC AML10). Blood.1997;89(7):2311-8.
- Estey EH, Thall PF, Cortes JE, et al. Comparison of idarubicin + ara-C-, fludarabine + araC-, and topotecan + ara-C-based regimens in treatment of newly diagnosed acute myeloid leukemia, refractory anemia with excess blasts in transformation, or refractory anemia with excess blasts. Blood. 2001;98(13):3575-83.
- Ossenkoppele GJ, Graveland WJ, Sonneveld P, et al. The value of fludarabine in addition to ARA-C and G-CSF in the treatment of patients with high-risk myelodysplastic syndromes and AML in elderly patients. Blood. 2004;103(8):2908-13.
- Milligan DW, Wheatley K, Littlewood T, Craig JIO, Burnett AK. Fludarabine and cytosine are less effective than standard ADE chemotherapy in high-risk acute myeloid leukemia, and addition of G-CSF and ATRA are not beneficial: results of the MRC AML-HR randomized trial. Blood. 2006;107(12):4614-22.
- List AF, Kopecky KJ, Willman CL, et al. Benefit of cyclosporine modulation of drug resistance in patients with poor-risk acute myeloid leukemia: a Southwest Oncology Group study. Blood. 2001;98(12):3212-20.
- Estey EH. Growth factors in acute myeloid leukaemia. Best Pract Res Clin Haematol. 2001;14(1):175-87.
- Löwenberg B, van Putten W, Theobald M, et al. Effect of priming with granulocyte colony-stimulating factor on the outcome of chemotherapy for acute myeloid leukemia. N Engl J Med. 2003;349(8):743-52.
- Thomas X, Raffoux E, de Botton S, et al. Effect of priming with granulocyte-macrophage colony-stimulating factor in younger adults with newly diagnosed acute myeloid leukemia: a trial by the Acute Leukemia French Association (ALFA) Group. Leukemia. 2007;21(3):453-61.
- Büchner T, Berdel WE, Hiddemann W. Priming with granulocyte colony stimulating factor - relation to high-dose cytarabine in acute myeloid leukemia [comment]. N Engl J Med. 2004;350(21):2215-6.
- Mayer RJ, Davis RB, Schiffer CA, et al. Intensive post-remission chemotherapy in adults with acute myeloid leukemia. N Engl J Med.1994;331(14):896-903.
- Bloomfield CD, Lawrence D, Byrd JC, et al. Frequency of prolonged remission duration after high-dose cytarabine intensification in acute myeloid leukemia varies by cytogenetic subtype. Cancer Res. 1998;58(18):4173-9.
- Elonen E, Almqvist A, Hänninen A, et al. Comparison between four and eight cycles of intensive chemotherapy in adult acute myeloid leukemia: a randomized trial of the Finnish Leukemia Group. Leukemia. 1998;12(7):1041-8.
- Burnett AK, Goldstone AH, Stevens RMF, et al. Randomized comparison of addition of autologous bone-marrow transplantation to intensive chemotherapy for acute myeloid leukaemia in first remission: results of MRC AML 10 trial. Lancet.1998;351(9104):700-8.
- Stone RM, Berg DT, George SL, et al. Post-remission therapy in older patients with de novo acute myeloid leukemia: a randomized trial comparing mitoxantrone and intermediate-dose cytarabine with standard-dose cytarabine. Blood. 2001;98(3):548-53.
- Büchner T, Hiddemann W, Berdel WE, et al. 6-thioguanine, cytarabine, and daunorubicin (TAD) and high-dose cytarabine and mitoxantrone (HAM) for induction, TAD for consolidation, and either prolonged maintenance by reduced monthly TAD or TAD-HAM-TAD and one course of intensive consolidation by sequential HAM in adult patients at all ages with de novo acute myeloid leukemia (AML): a randomized trial of the German AML Cooperative Group. J Clin Oncol. 2003;21(24):4496-504.
- Weisser M, Hafelach C, Hiddermann W, Schittgers S. The quality of molecular response to chemotherapy is predictive for outcome of AML1-ETO positive AML and is independent of pretreatment risk factors. Leukaemia. 2007;21(6);1771-82.
- Schlenk RF, Fröhling S, Hartmann F, et al. Intensive consolidation versus oral maintenance therapy in patients 61 years or older with acute myeloid leukemia in first remission: results of second randomization of the AML HD98-B treatment trial [letter]. Leukemia. 2006;20(4):748-50.
- Latagliata R, Breccia M, Fazi P, et al. Liposomal daunorubicin versus standard daunorubicin: long term follow-up of the GIMEMA GSI 103 AMLE randomized trial in patients older than 60 years with acute myelogenous leukaemia. Br J Haematol. 2008;143(5):681-9.
- Lim WS, Tardi PG, Dos Santos N, et al. Leukaemia-selective uptake of CPX-351, a synergistic fixed ratio cytarabine:daunorubicin formulation in bone marrow xenografts. Leuk Res. 2010;34(9):1214-23.
- Kim HP, Gerhard B, Harasym TO, Mayer LD, Hogge DE. Liposomal encapsulation of a synergistic molar ration of cytarabine and daunorubicin enhances selected toxicity for acute myeloid leukaemia progenitors compared to component analogue normal haemopoetic cells. Exp Hematol. 2011;39(7):741-50.
- O’Brien S, Rizzieri DA, Vey N, et al. A phase II multicentre study with elecytarabine as secondary slavage therapy in patients with AML. J Clin Onc. 2009; 27:15s:(suppl; abstr 1042).
- van Der Velden VH, te Marvelde JG, Hoogeveen PG, et al. Targeting of the CD33-calicheamicin immunoconjugate Mylotarg (CMA- 676) in acute myeloid leukemia: in vivo and in vitro saturation and internalization by leukemic and normal myeloid cells. Blood. 2001;97(10):3197-204.
- Sievers EL, Larson RA, Stadtmauer EA, et al. Efficacy and safety of gemtuzumab ozogamicin in patients with CD33-positive acute myeloid leukemia in first relapse. J Clin Oncol. 2001;19(13):3244-54.
- Kell WJ, Burnett AK, Chopra R, et al. A feasibility study of simultaneous administration of gemtuzumab ozogamicin with intensive chemotherapy in induction and consolidation in younger patients with acute myeloid leukemia. Blood. 2003;102(13):4277-83.
- Stasi R, Evangelista ML, Buccisano F, et al. Gemtuzumab ozogamicin in the treatment of acute myeloid leukemia. Cancer Treat Rev. 2008;34:49-60.
- Sievers EL, Appelbaum FR, Spielberger RT, et al. Selective ablation of acute myeloid leukemia using antibody- targeted chemotherapy: A phase I study of an anti-CD33 calicheamicin immunoconjugate. Blood. 1999;93:3678-84.
- Karp JE, Gojo I, Pili R, et al. Targeting vascular endothelial growth factor for relapsed and refactory adult acute myelogenous leukaemia. Clin Cancer Res. 2004;10(11):3577-85.
- Weisberg E, Boulton C, Kelly LM, et al. Inhibition of mutant FLT3 receptors in leukemia cells by the small molecule tyrosine kinase inhibitor PKC412. Cancer Cell. 2002;1(5):433-43.
- Levis M, Allebach J, Tse KF, et al. A FLT3-targeted tyrosine kinase inhibitor is cytotoxic to leukemia cells in vitro and in vivo. Blood. 2002;99(11):3885-91.
- O’Farrell A-M, Abrams TJ, Yuen HA, et al. SU11248 is a novel FLT3 tyrosine kinase inhibitor with potent activity in vitro and in vivo. Blood. 2003;101(9):3597-3605.
- Stone RM, DeAngelo DJ, Klimek V, et al. Patients with acute myeloid leukemia and an activating mutation in FLT3 respond to a small-molecule FLT3 tyrosine kinase inhibitor, PKC412. Blood. 2005;105(1):54-60.
- Smith BD, Levis M, Beran M, et al. Single-agent CEP-701, a novel FLT3 inhibitor, shows biologic and clinical activity in patients with relapsed or refractory acute myeloid leukemia. Blood. 2004;103(10):3669-76.
- Heidel F, Cortes J, Rucker FG, et al. Results of a multi-centre phase II trial for older patients with c-KIT-positive acute myeloid leukaemia and high-risk myelodysplastic syndrome using low-dose Ara-c and Imatinib. Cancer. 2007;109(5):907-14.
- Giles FJ, Bellamy WT, Estrov Z, et al. The anti-angiogenesis agent, AG- 013736, has minimal activity in elderly patients with poor prognosis acute myeloid leukaemia or myelodysplastic syndrome. Leuk Res. 2006;30(7):801-11.
- Yee KE, Zeng Z, Konopleva M, et al. Phase I/II study of the mammalian target of rapamycin inhibitor everolimus in patients with relapsed or refractory hematological malignancies. Clin Cancer Res. 2006;12(17):5165-73.
- Rizzieri DA, Feldman E, Dipersio JF, et al. A phase 2 clinical trial of deforolimus, a novel mammalian target of rapamycin inhibitor, in patients with relapsed or refractory hematological malignancies. Clin Cancer Res. 2008;14(9):2752-
- Karp JE, Flatten K, Feldman EJ, et al. Active oral regimen for elderly adults with newly diagnosed acute myelogenous leukaemia: a preclinical and phase I trial of tipifarnib combined with etoposide. Blood. 2009;113(20):4841-52.
- Ravoet C, Mineur P, Robin V, et al. Farnesyl transferase inhibitor (lonofarnib) in patients with myelodysplastic syndrome or secondary acute myeloid leukaemia: a phase II study. An Hematol. 2008;87(11):881-5.
- Cortes J, Faderl S, Estey E, et al. A phase I study of BMS-214662, a farnesyl transferase inhibitor in patients with acute leukaemias and myelodysplastic syndromes. J Clin Oncol. 2005;23(12):2805-12.
- Fiedler W, Serve H, Döhner H, et al. A phase I study of SU11248 in the treatment of patients with refractory or resistant acute myeloid leukemia (AML) or not amenable to conventional therapy for the disease. Blood. 2005;105(3):986-93.
- Silverman LR, Demakos EP, Peterson BL, et al. Randomized controlled trial of azacitidine in patients with the myelodysplastic syndrome: a study of the Cancer and Leukemia Group B. J Clin Oncol. 2002;20(10):2429-40.
- Wijermans P, Lübbert M, Verhoef G, et al. Low-dose 5-aza-2- deoxycytidine, a DNA hypomethylating agent, for the treatment of high-risk myelodysplastic syndrome: a multicenter phase II study in elderly patients. J Clin Oncol. 2000;18(5):956-62.
- Issa J-P, Garcia-Manero G, Giles FJ, et al. Phase 1 study of low-dose prolonged exposure schedules of the hypomethylating agent 5-aza- 2-deoxycytidine (decitabine) in hematopoietic malignancies. Blood. 2004;103(5):1635-40.
- Fenaux P, Mufti GJ, Hellstrom-Lindberg E, et al. Efficacy of azacitidine compared with that of conventional care regimens in the treatment of higher-risk myelodysplastic syndromes: a randomised, open-label, phase III study. Lancet Oncol. 2009;10(3):223-32.
- Burnett AK, Baccarani M, Johnson P. Effectiveness of clofarabine in elderly AML patients with adverse cytogenetics unfit for intesive chemotherapy. Blood. 2006;108;1985a.
- Burnett AK, Russell NH, Kell J, et al. European develpment of Clofarabine as treatment for older patients considered unsuitable for induction chemotherapy. J Clin Onc. 2010;28:2389-95.
- Giles FJ, Cortes JE, Baker SD, et al. Troxacitabine, a novel dioxolone nucleosidase analogue, has activity in patients with advanced leukaemia. J Clin Oncol. 2001;19:762-71.
- Roboz GJ, Giles FJ, Ritchie EK, et al. Phase I/II study of continuous infusion Troxacitabine in refractory AML. J Clin Oncol. 2006;25:10-15.
- Garcia-Manero G. Luger S, Vemigopal P, et al. A randomized phase II study of sapacitabine in elderly patients with AML previously untreated or in first relapse or previously treated MDS. J Clin Oncol. 2009; 27:15s.(Suppl;abstr. 7021).
- Giles FJ, Stock W, Vey N, et al. A double-blind placebo-controlled randomised phase III study of high dose araC with or without cloretazine in patients with first relapse of acute myeloid leukaemia. Blood. 2006; 108:(Suppl, abstr. 1970).
- Yang LP, Perry CM. Histamine dihydrochloride in the management of acute myeloid leukaemia. Drugs. 2011;71(1):109-22.
- Bug G, Ritter M, Wassmann B, et al. Clinical trial of valproic acid and all-trans retinoic acid in patients with poor-risk acute myeloid leukemia. Cancer. 2005;104(12):2717-25.
- Raffoux E, Cras A, Recher C, et al. Phase 2 clinical trial of Azacetidine, valproic acid and all trans retinoic acid in patients with high-risk acute myeloid leukemia or myelodysplastic syndrome. Oncotarget. 2010;1(1):34-42.
- Burnett AK, Milligan D, Goldstein A, et al. The impact of dose escalation and resistance modulation in older patients with acute myeloid leukaemia and high risk myelodysplastic syndrome: the results of the LRF AML14 trial. Br J Haematol. 2009;145(3):318-32.
- Lancet JE, Baer MR, Duran GE , et al. A phase I trial of continuous infusion of the multidru resistant inhibitor zosuquidar with duanorubicin and cytarabine in acute myeloid leukaemia. Leuk Res. 2009;33(8):1055-61.
- Schmitt M, Casalegno-Garduño R, Xu X, Schmitt A. Peptide vaccines for patients with acute myeloid leukemia. Expert Rev Vaccines. 2009;8(10):1415-25.
- Qazilbash MH, Wieder E, Rios R, et al.Vaccination with the PR-1 leukemia-associated antigen can induce complete remission in patients with myeloid leukemia. Blood. 2004;104:259 (Abstract).
- Qazilbash MH, Wieder E, Thall PF, et al. PR-1 vaccine elicited immunological response after hematopoietic stem cell transplantation is associated with better clinical response event-free survival. Blood. 2007;110:577 (abstract).
- Schmitt M, Schmitt A, Rojewski M, et al.RHAMM-R3 peptide vaccination in patients with acute myeloid leukemia, myelodysplastic syndrome and multiple myeloma elicits immunological and clinical responses. Blood. 2008;11:1357-65.
- Giannopoulos K, Kowal M, Dmoszynska A, et al.Peptide vaccination induces dynamic changes in CD4^^ and CD8^^T cell subsets: report on the first peptide vaccination trial in patients with chronic lymphocytic leukemia (CLL). Blood. 2008;112:3159 (abstract).
- Greiner J, Schmitt A, Giannopoulos K, et al.High-dose RHAMM-R3 peptide peptide vaccination for patients with vaccination for patients with acute myeloid leukemia (AML), myelodysplastic syndrome (MDS), multiple myeloma (MM) and chronic lymphocytic leukemia (CLL). Blood. 2008;112:2911(abstract).
- Keilholz U, Letsch A, Busse A, et al. A clinical and immunologic Phase 2 trial of Wilms tumor gene product 1 (WT-1) peptide vaccination in patients with AML and MDS. Blood. 2009;113:6541-8.
- Mailander V, Scheibenbogen C, Thiel E, et al. Complete remission in a patient with recurrent acute myeloid leukemia induced by vaccination with WT-1 peptide in the absence of haematological or renal toxicity. Leukemia. 2004;18:165-6.
- Oka Y, Tsuboi A, Taguchi T, et al. Induction of WT-1 (Wilms’ tumor gene)-specific cytotoxic T lymphocytes by WT-1 peptide vaccine and the resultant cancer regression. Proc. Natl Acad. Sci. USA. 2004;101:13885-90.
- Rezvani K. PR-1 vaccination in myeloid malignancies. Expert Rev. Vaccines. 2008;7(7):867-75.
- Rezvani K, Yong AS, Mielke S, et al.Leukemia-associated antigenspecific T-cell responses following combined PR-1 and WT-1 peptide vaccination in patients with myeloid malignancies. Blood. 2008;111:236-42.
- Wagner EM, Kuball J, Wattad M, Huber C, Heit W, Theobald M. Vaccination with WT-1 and PR-3 derived peptides in patients with AML/MDS and MUC1 peptides in patients with multiple myeloma - preliminary results. Blood. 2008;108:4582 .
- Brenner MK. The contribution of marker gene studies to hemopoietic stem cell therapies. Stem Cells. 1995;13(5):453-61.
- Jacob H, Hube A. Preservation of stem cells. Organogenesis. 2009;5(3):134-7.
- 112.Riggs JR, Wanta SM, Lekic N, Craig MJ, Gallicano GI.Current and prospectivetherapies to treat leukaemia. Journal of Hematological Malignancies. 2011;1(1):24-34.
- Slovak ML, Kopecky KJ, Cassileth PA, et al. Karyotypic analysis predicts outcome of pre-remission and post-remission therapy in adult acute myeloid leukemia: a Southwest Oncology Group/Eastern Cooperative Oncology Group study. Blood. 2000;96(13):4075-83.
- Schlenk RF, Benner A, Hartmann F, et al. Risk adapted post-remission therapy in acute myeloid leukemia: results of the German Multicenter AML HD93 treatment trial. Leukemia. 2003;17(8):1521-8.
- Breems DA, Löwenberg B. Acute myeloid leukemia and the position of autologous stem cell transplantation. Semin Hematol. 2007;44(4):259-66.
- Whitman SP, Ruppert AS, Marcucci G, et al. Long-term disease-free survivors with cytogenetically normal acute myeloid leukemia and MLL partial tandem duplication: a Cancer and Leukemia Group B study. Blood. 2007;109(12):5164-7.
- Horowitz MM, Gale RP, Sondel PM. Graft-versus-leukemia reactions after bone marrow transplantation. Blood. 1990;75(3):555-62.
- Cornelissen JJ, van Putten WLJ, Verdonck LF, et al. Results of a HOVON/SAKK donor versus no-donor analysis of myeloablative HLA-identical sibling stem cell transplantation in first remission acute myeloid leukemia in young and middle-aged adults: benefits for whom? Blood. 2007;109(9):3658-66.
- Yanada M, Matsuo K, Emi N, Naoe T. Efficacy of allogeneic hematopoietic stem cell transplantation depends on cytogenetic risk for acute myeloid leukemia in first disease remission: a metaanalysis. Cancer. 2005;103(8):1652-8.
- Koreth J, Schlenk R, Kopecky KJ, et al. Allogeneic stem cell transplantation for acute myeloid leukemia in first complete remission: systematic review and meta-analysis of prospective clinical trials. JAMA. 2009;301(22):2349 61.
- Reiffers J, Gaspard MH, Maraninchi D, et al. Comparison of allogeneic or autologous bone marrow transplantation and chemotherapy in patients with acute myeloid leukaemia in first remission. a prospective controlled trial. Br J Haematol. 1989;72:57.
- Lowenberg B, Verdonck LJ, Dekker AW, et al. Autologous bone marrow transplantation in acute myeloid leukemia in first remission: results of a Dutch prospective study. Journal of Clinical Oncology.1990;8(2) 287-94.
- Zittoun RA, Mandelli F, Willemze R, et al.Autologous or allogeneic bone marrow transplantation compared with intensive chemotherapy in acute myelogenous leukemia. European Organization for Research and Treatment of Cancer (EORTC) and the Gruppo Italiano Malattie Ematologiche Maligne dell’Adulto (GIMEMA). N Engl J Med.1995;332(4):217-23.
- Harousseau JL, Cahn J, Pignon B, et al. Comparison of autologous bone marrow transplantation and intensive chemotherapy as postremission therapy in adult acute myeloid leukaemia. The Groupe Ouest Est Leuce´mies Aigue¨s Mye´loblastiques (GOELAM). Blood. 1997;90:2978-86.
- Cassileth PA, Harrington DP, Appelbaum FR, et al. Chemotherapy compared with autologous or allogeneic bone marrow transplantation in the management of acute myeloid leukemia in first remission. N Engl J Med.1998;339(23):1649-56.
- Burnett AK, Goldstone AH, Stevens RM, et al. Randomised comparison of addition of autologous bone-marrow transplantation to intensive chemotherapy for acute myeloid leukaemia in first remission:results of MRC AML 10 trial. Lancet. 1998;351:700.
- Blume KG, Thomas ED. A Review of Autologous Hematopoietic Cell Transplantation. Bio Blood Marrow Transplant. 2000;6(1):1-12.
- Ortega JJ, Diaz de Heredia C, Olive T, et al. Allogeneic and autologous bone marrow transplantation after consolidation therapy in high-risk acute myeloid leukemia in children. Towards a riskoriented therapy. Haematologica. 2003;88:290-9.
- Woods WG, Neudorf S, Gold S, et al. A comparison of allogeneic bone marrow transplantation, autologous bone marrow transplantation, and aggressive chemotherapy in children with acute myeloid leukemia in remission. Blood. 2001;97:56-62.
- Stevens RF, Hann IM, Wheatley K, Gray RG. Marked improvements in outcome with chemotherapy alone in paediatric acute myeloid leukemia: results of the United Kingdom Medical Research Council’s 10thAML trial. MRC Childhood Leukaemia Working Party. Br J Haematol.1998;101:130-40.
- Alonzo TA, Wells RJ, Woods WG, et al. Postremission therapy for children with acute myeloid leukemia: the children’s cancer group experience in the transplant era. Leukemia. 2005;19:965-70.
- Reinhardt D, Kremens B, Zimmermann M, et al. No improvement of overall-survival in children with high-risk acute myeloid leukemia by stem cell transplantation in 1st complete remission. Blood. 2006;108:99a.
- Klingebiel T, Reinhardt D, Bader P. Place of HSCT in treatment of childhood AML. Bone Marrow Transplantation. 2008;42:S7-S9.
- Schetelig J, Bornhäuser M, Schmid C, et al. Matched unrelated or matched sibling donors result in comparable survival after allogeneic stem-cell transplantation in elderly patients with acute myeloid leukemia: a report from the Cooperative German Transplant Study Group. J Clin Oncol. 2008;26(32):5183-91.
- Boglarka Gyurkocza, Frederick R. Appelbaum. Identifying Older Patients With Acute Myeloid Leukemia Who May Be Candidates for Reduced-Intensity Hematopoietic Cell Transplantation. Natl Compr Canc Netw. 2011(9):319-30.
- Lee SE, Lim J, Yahng SA, et al. Reduced-intensity conditioning regimen combined with low-dose total body irradiation in the treatment of myelodysplastic syndrome. Acta Haematol. 2011;126(1):21-9.
- Patel P, Saraf S, Rondelli D. Allogenic stem cell transplantation to cure acute myeloid leukaemia in elderly patients. Journal of Advances in Internal Medicine. 2012;1(1):43-9.
- Morecki S, Yacovlev E, Gelfand Y, Shabat Y, Slavin S .Induction of graft-versus-leukemia (GVL) effect without graft-versus-host disease (GVHD) by pretransplant donor treatment with immunomodulators. Biol Blood Marrow Transplant. 2009;(15):406-15.
- Brunstein CG, Gutman JA, Weisdorf DJ, et al. Allogeneic hematopoietic cell transplantation for hematological malignancy: relative risks and benefits of double umbilical cord blood. Blood. 2010;116(22):4693-9.
- Janus A, Robak T, Smolewski P. The mammalian target of the rapamycin (mTOR) kinase pathway: its role in tumourigenesis and targeted antitumour therapy. Cell Mol Biol Lett. 2005;10(3):479-98.
- Park S, Chapuis N, Tamburini J, et al. Role of PI3K/AKT and mTOR signalling pathwys in acute myeloid leukaemia. Haematologica. 2010;95(5):819-28.
- Gills JJ, Dennis PA. Perifosine: update on a novel AKT inhibitor. Curr Oncol Rep. 2009;11(2):102-110.
- Crul M, Rosing H, de Klerk, et al. Phase I and pharmacological study of daily oral administration of perifosine in patients with advanced solid tumours. Eur J Cancer. 2002;38(12):1615-21.
- Fiegl M, Lindner LH, Juergens M, Eibl H, Hiddemann W, Braess J. Erofusine, a novel alkylphosphocholine, in acute myeloid leukaemia: single activity and combination with other antileukaemic drugs. Cancer Chemother Pharmacol. 2008;62(2):321-9.
- Agrawal-Singh S, Isken E, Agelopoulos K, et al. Genome wide analysis of histone H3 acetylation patterns in AML identifies PRDX2 as an epigentically silenced tumor suppressor gene. Blood. 2012;119(10):2346-57.
- Langemeijer SM, Kuiper RP, Berends M, et al. Acquired mutations in TET2 are common in myelodysplastic syndromes. Nat Genet. 2009;41(7):838-42.
- Tefferi A, Lim KH, Levine R. Mutation in TET2 in myeloid cancers. N Engl J Med. 2009;361(11):1117.
- Nibourel O, Kosmider O, Cheok M, et al. Incidence and prognostic value of TET2 alterations in de novo acute myeloid leukemia achieving complete remission. Blood. 2010;116(7):1132-5.
- Figueroa ME, Abdel-Wahab O, Lu C, et al. Leukemic IDH1 and IDH2 mutations result in a hypermethylation phenotype, disrupt TET2 function, and impair hematopoietic differentiation. Cancer Cell. 2010;18(6):553-67.
- Ito S, D’Alessio AC, Taranova OV, Hong K, Sowers LC, Zhang Y. Role of Tet proteins in 5mC to 5hmC conversion, ES-cell self-renewal and inner cell mass specification. Nature. 2010;466(7310):1129-33.
- Moran-Crusio K, Reavie L, Shih A, Abdel-Wahab O, Ndiaye-Lobry D, Lobry C, et al. Tet2 loss leads to increased hematopoietic stem cell self-renewal and myeloid transformation. Cancer Cell. 2011;20(1):11- 24.
- Li Z, Cai X, Cai CL, Wang J, Zhang W, Petersen BE, et al. Deletion of Tet2 in mice leads to dysregulated hematopoietic stem cells and subsequent development of myeloid malignancies. Blood. 2011;118(17):4509-18.
- Quivoron C, Couronne L, Della Valle V, Lopez CK, Plo I, Wagner- Ballon O, et al. TET2 inactivation results in pleiotropic hematopoietic abnormalities in mouse and is a recurrent event during human lymphomagenesis. Cancer Cell. 2012;20(1):25-38.
- Weissman S, Alpermann T, Grossmann V, et al. Landscape of TET2 Mutations in acute myeloid leukaemia. Leukemia. 2011;Nov25:doi:10.1038/leu.2011.326.
- Chou W-C, Chou S-C, Liu C-Y, et al. TET2 mutation is an unfavourable prognostic factor in acute myeloid leukemia patients with intermediate risk cytogenetics. Blood. 2011;118(14):3803-10.
- Kosmider O, Delabesse E, Mansat-De Mas V, et al. TET2 mutations in secondary acute myeloid leukemias: a French retrospective study. Haematologica. 2011;96(7):1059-63.
- Gaidzik VI, Paschka P, Spath D, et al. TET2 Mutations in Acute Myeloid Leukemia (AML): Results from a comprehensive genetic and clinical analysis of the AML Study Group. J Clin Oncol. 2012, March19:doi: 10.1200/JCO.2011.39.2886.
- Itzykson R, Kosmider O, Cluzeau T, et al. Impact of TET2 mutations on response rate to azacitidine in myelodysplastic syndromes and low blast count acute myeloid leukemias. Leukemia. 2011;25(7):1147- 52.
- Ley TJ, Ding L, Walter MJ, et al. DNMT3A mutations in acute myeloid leukemia. N Engl J Med. 2010;363(25):2424-33.
- Yan XJ, Xu J, Gu ZH, et al. Exome sequencing identifies somatic mutations of DNA methyltransferase gene DNMT3A in acute monocytic leukemia. Nat Genet. 2011;43(4):309-15.
- Shen Y, Zhu YM, Fan X, et al. Gene mutation patterns and their prognostic impact in a cohort of 1185 patients with acute myeloid leukemia. Blood. 2011;118(20):5593-603.
- Thol F, Damm F, Ludeking A, et al. Incidence and prognostic influence of DNMT3A mutations in acute myeloid leukemia. J Clin Oncol. 2011;29(21):2889-96.
- Vire E, Brenner C, Deplus R, Blanchon L, Fraga M, Didelot C, et al. The Polycomb group protein EZH2 directly controls DNA methylation. Nature. 2006;439(7078):871-4.
- Cedar H, Bergman Y. Linking DNA methylation and histone modification: patterns and paradigms. Nat Rev Genet. 2009;10(5):295-304.
- Bruserud Ø, Gjertsen BT, Foss B, Huang T. New Strategies in the Treatment of Acute Myelogenous Leukemia (AML): In Vitro Culture of AML Cells---The Present Use in Experimental Studies and the Possible Importance for Future Therapeutic Approaches. Stem Cells. 2001;19:1-11.
- Bruserud Ø, Gjertsen BT, Brustugun OT, et al. Effects of interleukin 10 on blast cells derived from patients with acute myelogenous leukemia. Leukemia. 1995;9:1910-20.
- Blair A, Hogge DE, Sutherland HJ. Most acute myeloid leukemia progenitor cells with long-term proliferative ability in vitro and in vivo have the phenotype CD34+/CD71-/HLA-DR-. Blood. 1998;92:4325- 35.
- Nara N, McCulloch EA. The proliferation in suspension of the progenitors of the blast cells in acute myeloblastic leukemia. Blood. 1985;65:1484-93.
- Sutherland HJ, Blair A, Zapf RW. Characterization of a hierarchy in human acute myeloid leukemia progenitor cells. Blood. 1996;87:4754-61.
- Delwel R, Salem M, Pellens C, et al. Growth regulation of human acute myeloid leukemia: effects of five recombinant hematopoietic factors in a serum-free culture system. Blood. 1988;72:1944-49.
- Terpstra W, Ploemacher RE, Prins A et al. Fluorouracil selectively spares acute myeloid leukemia cells with long-term growth abilities in immunodeficient mice and in culture. Blood. 1996;88:1944-50.
- Bruserud Ø, Gjertsen BT, von Volkman HL. In vitro culture of human acute myelogenous leukemia (AML) cells in serumfree media; studies of native AML blasts and AML cell lines. J Hematother Stem Cell Res. 2000;9(6):923-32.
- Dick JE. Human stem cell assays in immunodeficient mice. Curr Opin Hematol. 1996;3:405-9.
- Blair A, Hogge DE, Ailles LE et al. Lack of expression of Thy-1 (CD90) on acute myeloid leukemia cells with long-term proliferative ability in vitro and in vivo. Blood. 1997;89:3104-12.
- Blair A, Hogge DE, Sutherland HJ. Most acute myeloid leukemia progenitor cells with long-term proliferative ability in vitro and in vivo have the phenotype CD34+/CD71-/HLA-DR-. Blood. 1998;92:4325- 35.
- Rombouts WJC, Martens ACM, Ploemacher RE. Identification of variables determining the engraftment potential of human acute myeloid leukemia in the immunodeficient NOD/SCIDhuman chimera model. Leukemia. 2000;14:889-97.
- Walter RB, Appelbaum FR, Tallman MS et al. Shortcomings in the clinical evaluation of new drugs: acute myeloid leukemia as paradigm. Blood. 2010;116(14):2420-28.
- Estey EH, Thall PF. New designs for phase 2 clinical trials. Blood. 2003;102(2):442-8.
- Rubinstein LV, Korn EL, Freidlin B, Hunsberger S, Ivy SP, Smith MA. Design issues of randomized phase II trials and a proposal for phase II screening trials. J Clin Oncol. 2005;23(28):7199-206.
- Berry DA. Bayesian clinical trials. Nat Rev Drug Discov. 2006;5(1):27- 36.
- Sonpavde G, Galsky MD, Hutson TE, Von Hoff DD. Patient selection for phase II trials. Am J Clin Oncol. 2009;32(2):216-9.
- Hunsberger S, Zhao Y, Simon R. A comparison of phase II study strategies. Clin Cancer Res. 2009;15(19):5950-5.
- Gail J. Roboz. Novel Approaches to the Treatment of Acute Myeloid Leukemia. Hematology Am Soc Hematol Educ Program.2011;1:43-50.
- Hassane DC, Guzman ML, Corbett C, et al. Discovery of agents that eradicate leukemia stem cells using an in silico screen of public gene expression data. Blood. 2008;111(12):5654-62.
- Lara PN, Jr., Higdon R, Lim N, et al. Prospective evaluation of cancer clinical trial accrual patterns: identifying potential barriers to enrollment. J Clin Oncol. 2001;19(6):1728-33.
- Dechartres A, Chevret S, Lambert J, Calvo F, Levy V. Inclusion of patients with acute leukemia in clinical trials: a prospective multicenter survey of 1066 cases. Ann Oncol. 2011;22(1):224-33.
- De Gruttola VG, Clax P, DeMets D et al. Considerations in the Evaluation of Surrogate Endpoints in Clinical Trials: Summary of a National Institutes of Health Workshop. Clin Trials. 2001;22:485-502.
- Swen JJ, Huizinga TW, Gelderblom H, et al. Translating Pharmacogenomics: Challenges on the Road to the Clinic. PLoS Med. 2007:4(8):e209