Keywords
|
Translational research; Alcohol addiction; Dopamine (DA); Phasic and tonic release; Ventral Tegmental Area (VTA); Nucleus accumbens; Medial prefrontal cortex; Insula, Optogenetics; Appetitive and consummatory behaviors |
Introduction
|
Alcohol is the most common drug of abuse in our society and, as a consequence, alcoholism is a devastating socio-economic problem estimated to account for 4% of the global burden of disease. Despite its widespread use and abuse, our understanding of the precise neural circuitry responsible for the development and progression of alcohol addiction is still incomplete. In fact, the successful development of more effective treatments will require a better understanding of the specific neurobiological mechanisms responsible for ethanol’s reinforcing actions and the pathological motivation to obtain the drug (despite serious negative consequences). Over the past several decades the development and use of translational animal models of addiction has resulted in remarkable progress in our understanding of ethanol’s acute effects on synaptic transmission and, perhaps more importantly, has provided critical insight into some of the neural circuits that control alcohol drinking behaviors. However, it is necessary to not only characterize the changes that manifest following alcohol exposure, but also to determine if these alterations play a causative role in the addiction process. Until recently, technical limitations have precluded many of the experiments that could establish the causal role of defined neural circuits in addiction-related behaviors. Specifically, these experiments would require the ability to manipulate discrete populations of neurons with precise temporal (ms) and spatial (μm) resolution in awake, behaving animals The development of optogenetics now provides researchers with the tools needed to control the activity of genetically defined neuronal circuits with light. Though alcohol researchers are only just beginning to use these tools, recent studies have already provided new insight into the causal role of specific neural circuits in alcohol addictionrelated behaviors. |
Neurochemical Changes Associated with Alcohol Exposure: Focus on Dopamine |
Researchers have long sought to elucidate the mechanisms of alcohol action in the brain. It is clear that ethanol affects brain function by modulating numerous neurotransmitter systems including but not limited to, GABA [1,2], glutamate [3], serotonin [4], norepinephrine [5], neuropeptide Y [6], vasopressin [7], adenosine [8] and dopamine (DA) [9-11]. Over the past twenty years, much attention has focused on the mesolimbic DA system, which has been strongly associated with the positive reinforcing effects of alcohol [9,10,12,13]. This circuit is comprised of the dopaminergic neurons in the ventral tegmental area (VTA) and their projections to the nucleus accumbens and several other brain regions. |
Rodent studies indicate that acutely administered alcohol increases the firing rate of VTA DA neurons [14], thereby elevating DA release in terminal fields like the nucleus accumbens [15,16], while DA uptake remains unchanged [17-19]. PET studies have shown that alcohol acutely promotes accumbal DA release in humans [20]. It is important to note that DA neurons exhibit two main firing modes: pacemaker, single spike activity at a frequency of ~5 Hz and bursts of action potentials at higher frequencies (≥20 Hz) [21,22]. These firing patterns generate distinct tonic and phasic patterns of DA release, resulting in DA concentration fluctuations on a time scale of several minutes and seconds, respectively [23]. These two modes of DA transmission appear to serve different functions that result in distinct behavioral consequences. Acute ethanol affects both tonic and phasic DA signaling, with the phasic response more pronounced in the nucleus accumbens [23]. Notably, the role of tonic and phasic DA transmission in ethanol drinking-related behaviors is unknown [24]. |
The consequences of chronic alcohol exposure on DA function are more complex. In general, there is evidence that chronic alcohol exposure and withdrawal lead to dopaminergic hypofunction, which may contribute to the maintenance of addiction [9,25]. In fact, low levels of DA have been reported in the caudate nucleus of deceased alcoholics [26]. In animal models of addiction, chronic administration of alcohol leads to substantial decreases in extracellular DA levels in the rat nucleus accumbens [27]. In addition, decreased expression of tyrosine hydroxylase and increased DA transporter (DAT) protein levels have been found in chronic ethanol-treated rats [28]. These findings suggest that decreased DA synthesis and enhanced DA uptake may contribute to the decline in extracellular DA concentrations associated with repeated ethanol exposure. Indeed, increased DA uptake rate has been found in the striatum of monkeys with a history of alcohol drinking [29], and in rats [30] and mice [31] chronically exposed to ethanol vapor. Chronic alcohol drinking may increase the sensitivity of the VTA to the reinforcing effects of ethanol [32,33] through increased excitation of DA neurons [34], a finding that may explain the greater accumbal DA release in response to acutely administered ethanol following chronic ethanol exposure [35]. Furthermore, chronic ethanol exposure may also change the sensitivity of presynaptic D2 DA receptors (D2R). These receptors are primarily involved in the control of DA release. The increased responsiveness of D2R was found in monkeys [29] and mice [31,36], but not in rats [30,37]. The transient nature of these changes, species differences in alcohol sensitivity and experimental protocols may account for these disparate findings. However, it should be highlighted that human alcoholics tend to have fewer D2Rs than nonalcoholics [38-41]. Currently, it is unknown whether the D2 receptor sensitivity is increased in the alcoholic brain. |
A great deal of research attention has focused on the role of DAergic signaling in the initiation of ethanol drinking (when there is no ethanol on board) and the actual behavior associated with the consumption of ethanol. The self-administration of drugs of abuse, like alcohol, is governed by appetitive and consummatory processes. Importantly, while both of these elements may play an integral role in the etiology of addiction, they are typically not correlated with each other and appear to be mediated by distinct neural circuits [42]. Several studies have attempted to clarify how accumbal DA influences appetitive and consummatory phases of alcohol intake [43]. For example, elegant microdialysis studies have shown a significant increase in accumbal DA during placement of rats into the operant chamber and in the first several minutes of ethanol consumption, while the motivated lever-pressing for ethanol did not produce an enhancement of DA levels in the nucleus accumbens. However, microdialysis is best suited to reveal changes in tonic DA activity that occur over minutes. Since accumbal DA concentrations fluctuate on both phasic (subsecond) and tonic (minutes) time scales in freely behaving rats, the relatively uniform DA concentrations obtained with microdialysis may mask changes in subsecond accumbal DA release. |
Therefore, there is now clear evidence from translational research that mesolimbic DA signaling can be profoundly influenced by acute and chronic alcohol. Moreover, intriguing but correlative data suggest that physiologically released DA may play an important role in ethanol drinking-related behaviors. Nevertheless, numerous questions regarding the precise role of DA release changes in alcohol addiction-related drinking behaviors remain unanswered. For example, the specific role of phasic and tonic DA signaling in appetitive and consummatory elements of ethanol drinking behaviors is poorly understood. Furthermore, the nucleus accumbens also receives strong glutamatergic innervation from areas like the prefrontal cortex, amygdala and insula, and the role of these excitatory inputs in ethanol self-administration and DA signaling is not clear. |
From Correlations to Causalities |
Assessing the neurochemical changes associated with alcohol drinking-related behaviors is important. However, the real challenge is to establish whether observed changes in neurotransmission actually play a causal role in the initiation or suppression of alcohol seeking and/or consumption. Optogenetics now allow us to answer these questions. This technique utilizes light to stimulate or inhibit the activity of discrete populations of neurons, including DA neurons [44-47], which have been genetically modified to express light-sensitive channels. Unlike traditional approaches, that employ electrical or chemical activation of DA neurons, optogenetics permit for the selective activation of DA neurons, without also affecting fibers of passage and non-DA neurons (e.g. GABAergic and cholingeric interneurons). Figure 1 demonstrates the precision by which DA can be released through optical stimulation of DA cell bodies in the midbrain [47]. Therefore, a far more precise exploration of the role of DA transmission in alcohol drinking behaviors is now possible. |
We have used this approach to selectively control VTA DA release in rats during voluntary alcohol drinking using an intermittent two-bottle choice procedure [48-50]. These techniques allowed us to explore the role of tonic and phasic patterns of DA release associated with ethanol intake. It is important to highlight that this intermittent two-bottle choice procedure, in which subjects have access to 20% ethanol and water on Monday, Wednesday and Friday (only water on remaining days) engenders relatively high levels of daily ethanol intake with approximately 25% of the daily intake consumed in the first 30 minutes of each drinking session. Our first experiments clearly demonstrated that ethanol drinking can be selectively influenced by distinct patterns of VTA DA cell activation without a significant effect on water intake. Specifically, a significant delay to the first ethanol lick and a decrease in the total amount of ethanol consumption were found when DA cell bodies were stimulated at a low frequency (5 Hz) during the first 10 minutes of a drinking session (Figure 2A and 2B). Similar stimulation had no effect when delivered in the home cage during the 10 min preceding ethanol drinking sessions. Low frequency stimulation shifts accumbal DA signaling to tonic mode, characterized by a small but prolonged increase in the extracellular DA concentration. These changes can diminish phasic DA release via a D2 DA autoreceptor-mediated feedback mechanism [13]. It has been proposed that phasic dopamine is needed for the ability of reward predictive cues to motivate behaviors directed toward receiving the reward [51]. Therefore, optogenetically forcing DA release into a tonic mode may prevent phasic signals triggered by the contextual cues associated with the drinking environment in the beginning of a session. However, no significant changes in drinking behaviors following phasic stimulation were observed in the study. This inability of phasic DA release to enhance ethanol drinking measures may have resulted from a celling effect. Because these rats were likely already highly motivated to consume ethanol, further optogenetic enhancement of phasic DA signaling could not increase alcohol drinking further. On the other hand, appetitive (motivational) and consummatory measures of alcohol drinking are not correlated [42]. For example, blockade of accumbal D2 DA receptors selectively inhibits leverpressing for ethanol (appetitive) but has no effect on ethanol consumption [52]. Therefore, shifting DA transmission in the nucleus accumbens into a phasic mode may primarily promote alcohol seeking behavior without changes in the consumption. |
Another group has recently employed elegant optogenetic strategies to identify neuronal circuits that may regulate alcohol intake despite aversive consequences, a diagnostic hallmark of alcohol addiction. Based on prior findings, these investigators focused on glutamatergic projections from the medial prefrontal cortex and insula to the nucleus accumbens core [53]. Using the intermittent alcohol drinking model described above, they first demonstrated that, after 3-4 months, ethanol drinking in this model becomes resistant to aversive consequences like adulteration of the ethanol solution with a bitter tastant or pairing alcohol drinking with a footshock. Using optogenetic approaches to selectively manipulate PFC and insula glutamatergic synapses in the nucleus accumbens, they demonstrated that inhibition of either of these inputs selectively reduced aversion-resistant ethanol intake, with minimal effects on drinking that was not associated with aversive consequences. Additional studies that combined ex vivo electrophysiology and optogenetics revealed that aversion-resistant drinking was associated with the appearance of a novel form of NMDA receptor-mediated synaptic transmission at these inputs, mediated by GRIN2c receptors, that was active at hyperpolarized potentials. Moreover, genetic knockdown of GRIN2c receptors in the nucleus accumbens selectively reduced aversion-resistant ethanol intake. Together, these studies provide the first experimental demonstration of the causal role of prefrontal cortical regions in the regulation of aversion-resistant alcohol intake. Moreover, Grin2c subunits contribute to a newly identified adaptation at prefrontal glutamatergic synapses onto medium spiny neurons in the nucleus accumbens core and this plasticity promotes aversion-resistant intake. Given that compulsive intake of alcoholic beverages despite negative economic, legal and physical consequences is considered a major obstacle to treatment of alcohol addiction in humans [54], these data highlight hyperpolarization-resistant NMDA receptor blockers as a potential novel target for the development of better treatments for alcohol addiction. |
Together, these first studies to use optogenetic techniques to probe alcohol intake have provided important new insight into the neural circuits that regulate recreational and abusive alcohol drinking behaviors. By demonstrating that the selective manipulation of defined populations of neurons or synaptic terminals can directly influence ethanol consumption, these experiments are beginning to define some of the neural circuits that may contribute to the development and progression of alcoholism. One important message the emerges from these recent findings is that current translational research should focus not only on the strength of neuronal signaling through these circuits but also on the patterns of activity within these circuits. For example, based on the many studies demonstrating that acute ethanol exposure increases accumbal DA levels, much research effort has been devoted to the development of pharmacological treatments that could decrease DA levels in this brain region. However, our recent optogenetic data clearly demonstrate that increases in specific patterns of DA signaling can actually decrease voluntary ethanol drinking. Optogenetics, which allows precise experimental manipulation of defined neural circuits, represents a powerful method to examine the behavioral sequel associated with physiological and pathophysiological stimulation patterns of neuronal activity. However, in order to leverage this technique, it must be integrated with other methods, like in vivo multiunit recording and real-time neurochemical monitoring [55] to precisely monitor neuronal firing patterns, neurotransmitter release and clearance following optogenetic manipulations. An integration of these complementary methods should be employed to explore other critical issues, such as establishing the specific circuitry underlying appetitive and consummatory alcoholdrinking behaviors and the neuroadaptations in these circuits that contribute to dependence-induced increases in ethanol drinking. Such studies will no doubt lead to the identification of many novel substrates that can be targeted for the development of more effective treatments for alcohol use disorders. |
Acknowledgements
|
Funded by NIH grants AA022449 and AA17531, and by the Russian Science Foundation grant N14-50-00069. |
Figures at a glance
|
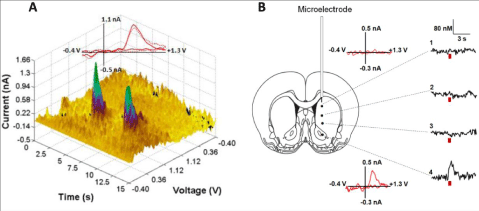 |
 |
Figure 1 |
Figure 2 |
|
|
|